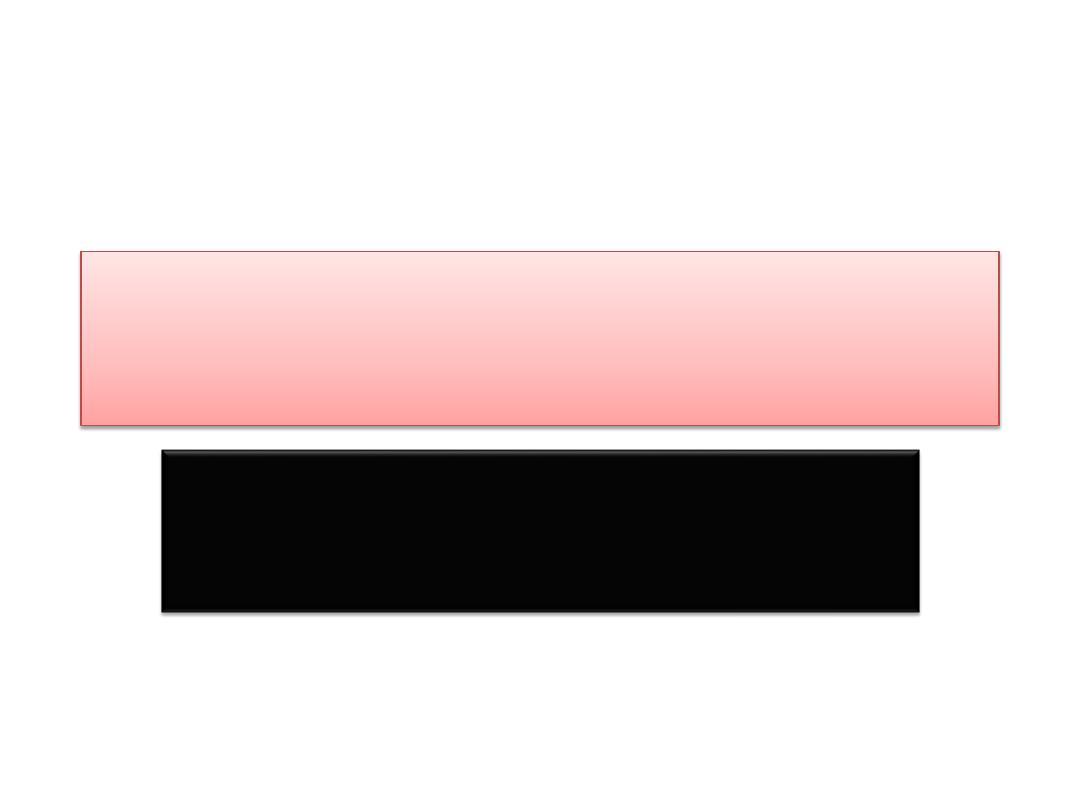
بسم ه الرحمن الرحيم
ACIDIFICATION OF THE URINE &
BICARBONATE EXCRETION
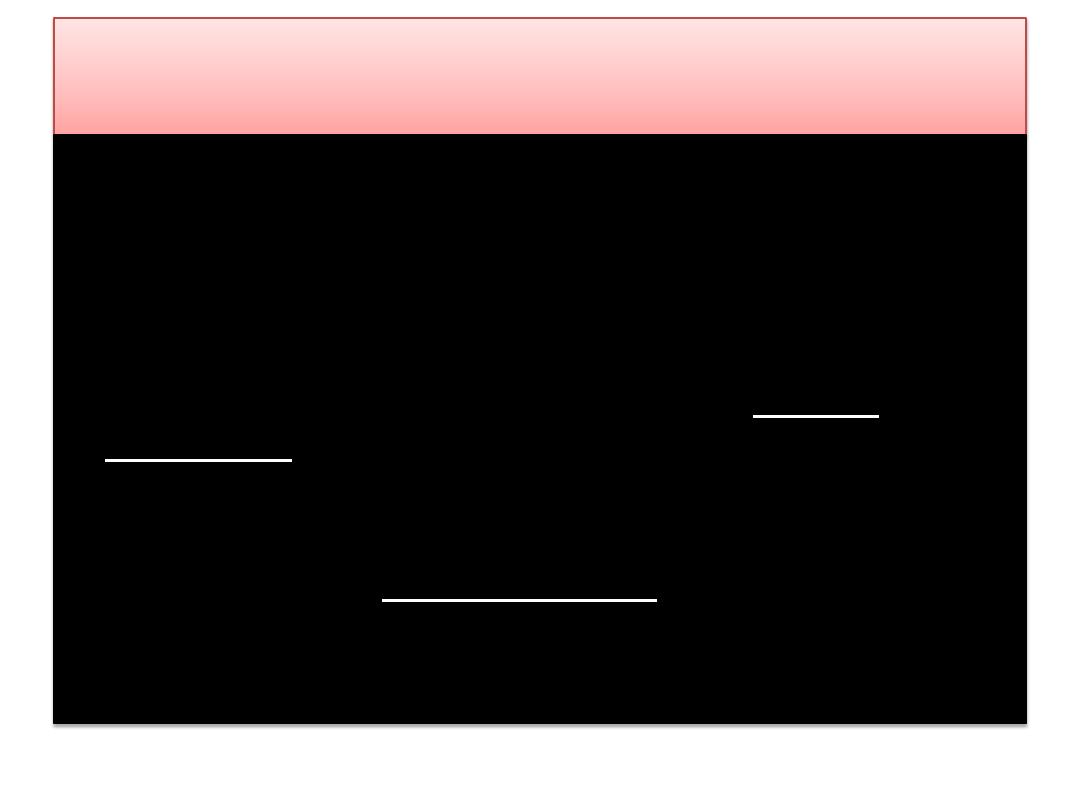
H
+
Secretion
• The cells of the
proximal
and
distal
tubules, like
the cells of the gastric glands,
secrete hydrogen
ions .
• Acidification also occurs in the
collecting
ducts.
• The reaction that is primarily responsible for H
+
secretion in the
proximal
tubules is Na
+
-H
+
exchange .
• This is an example of
secondary active transport
;
extrusion of Na
+
from the cells into the
interstitium by Na
+
-K
+
ATPase lowers intracellular
Na
+
, and this causes Na
+
to enter the cell from the
tubular lumen, with coupled extrusion of H
+
.
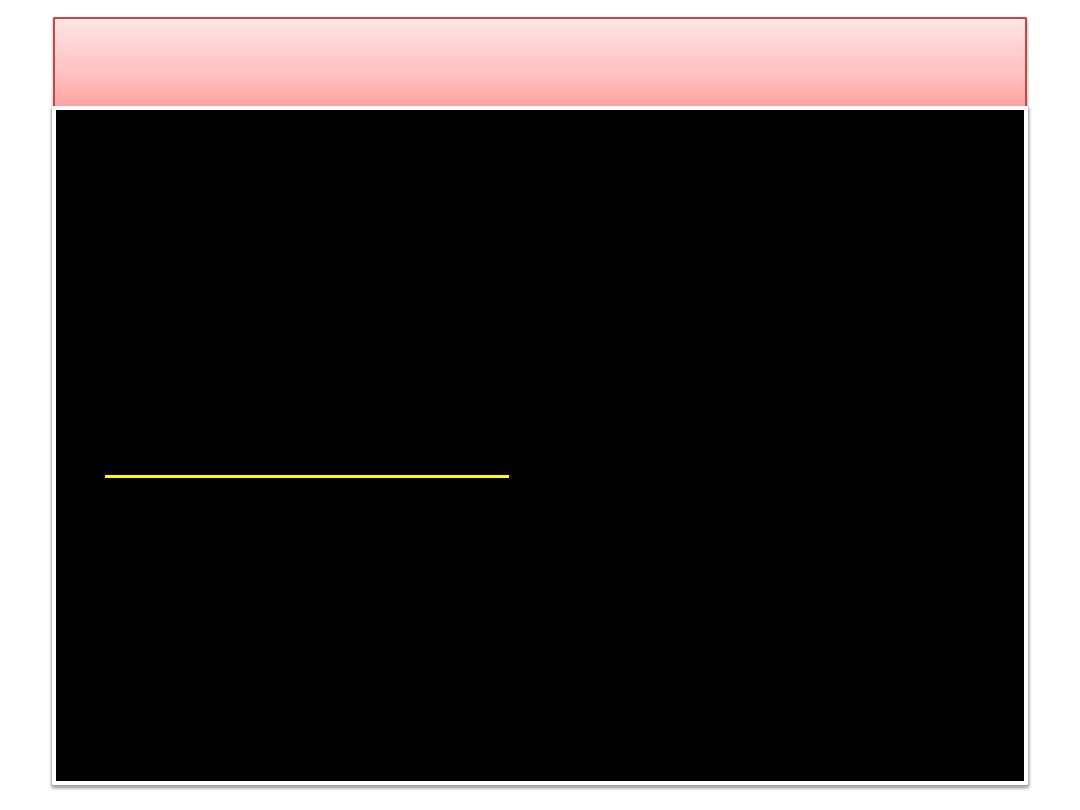
H
+
Secretion
•
+
The H
+
comes from
intracellular dissociation
of H
2
CO
3
, and the HCO
3
-
that is formed
diffuses
into the interstitial fluid.
• Thus, for
each H
+
ion secreted,
one Na
+
ion
and
one HCO
3
-
ion enter the interstitial fluid.
• Carbonic anhydrase
catalyzes the formation
of H
2
CO
3
, and
drugs
that inhibit carbonic
anhydrase depress both secretion of acid by
the
proximal tubules
and the reactions
which depend on it.
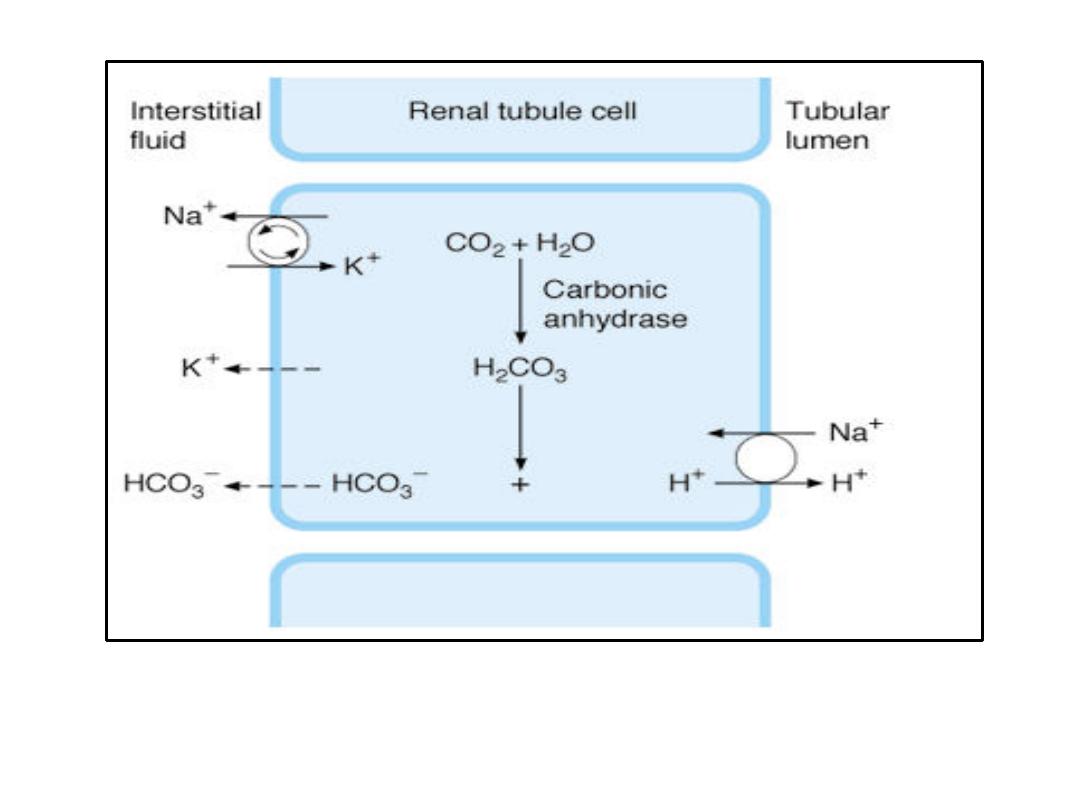
Secretion of acid by proximal tubular cells in the kidney. H
+
is transported
into the
tubular lumen by an antiport in exchange for Na
+
. Active transport by Na
+
-K
+
ATPase is indicated by arrows in the circle. Dashed arrows indicate diffusion.
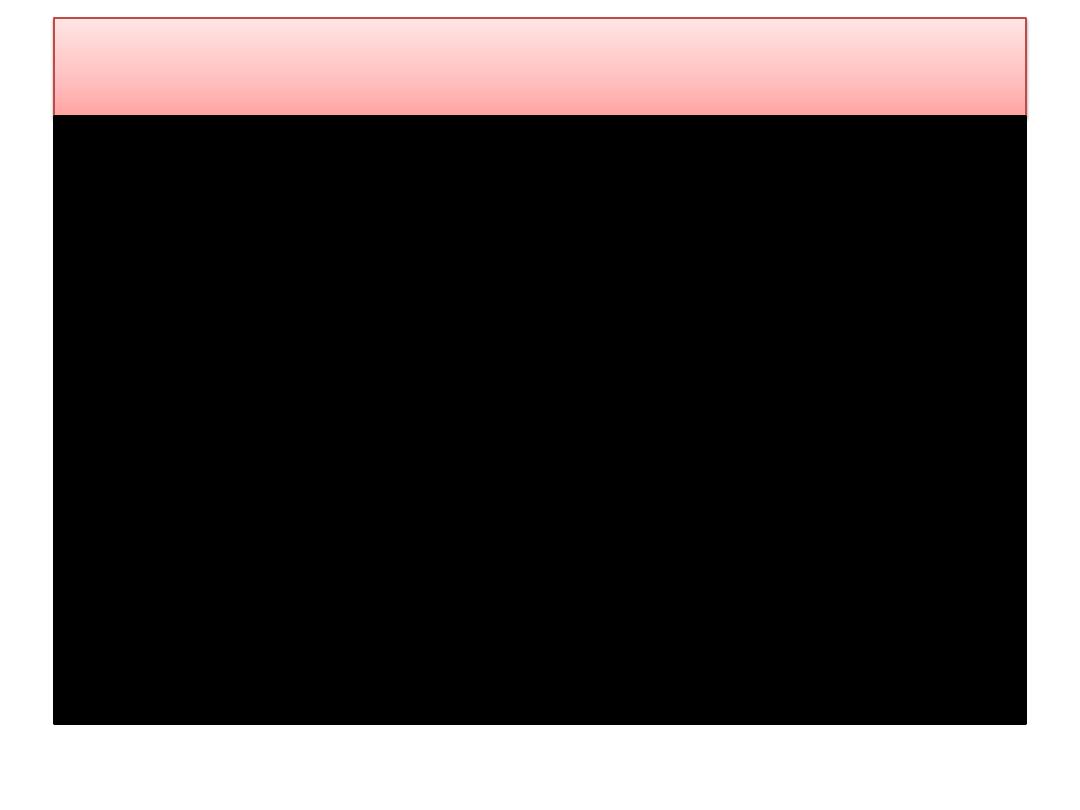
H
+
Secretion
• This is in contrast to what occurs in the distal
tubules and collecting ducts, where H
+
secretion is relatively
independent of Na
+
in
the tubular lumen.
• In this part of the tubule, most H
+
is secreted
by an
ATP-driven proton pump.
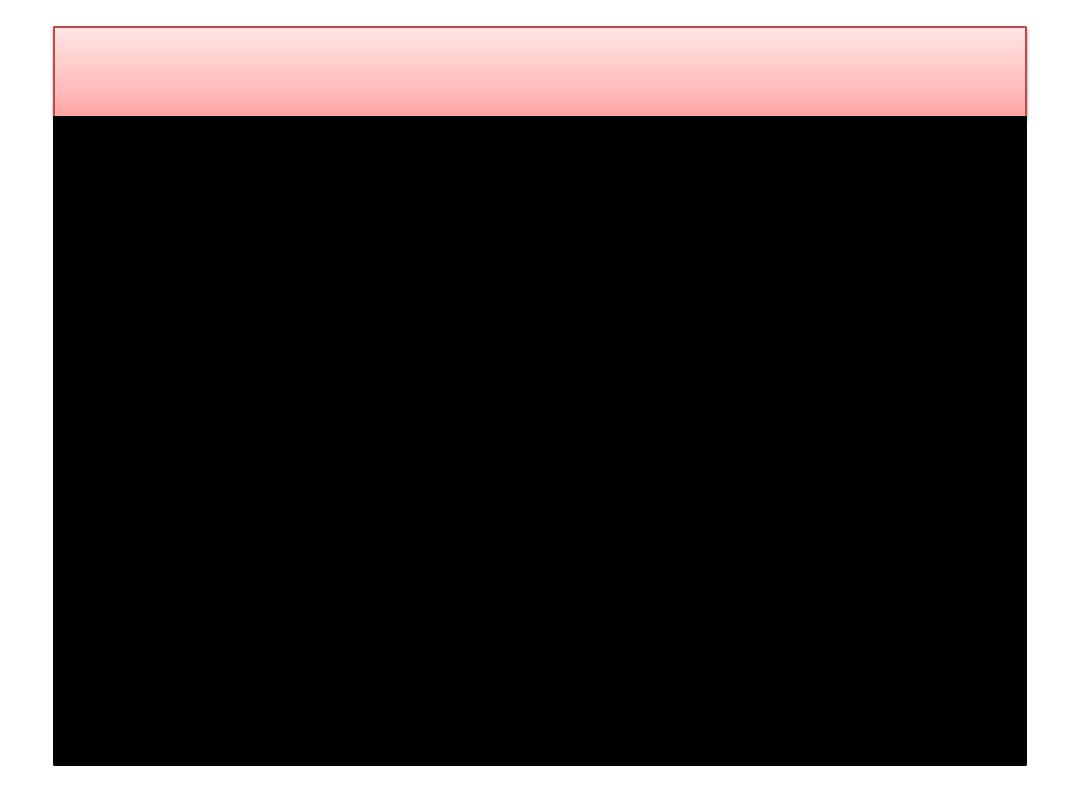
H
+
Secretion
• Aldosterone
acts on this pump to increase
distal H
+
secretion.
• The
I cells
in this part of the renal tubule
secrete acid and, like the parietal cells in
the stomach, contain abundant
carbonic
anhydrase
and numerous tubulovesicular
structures.
• Some of the H
+
is also secreted by
H
+
-K
+
ATPase
.
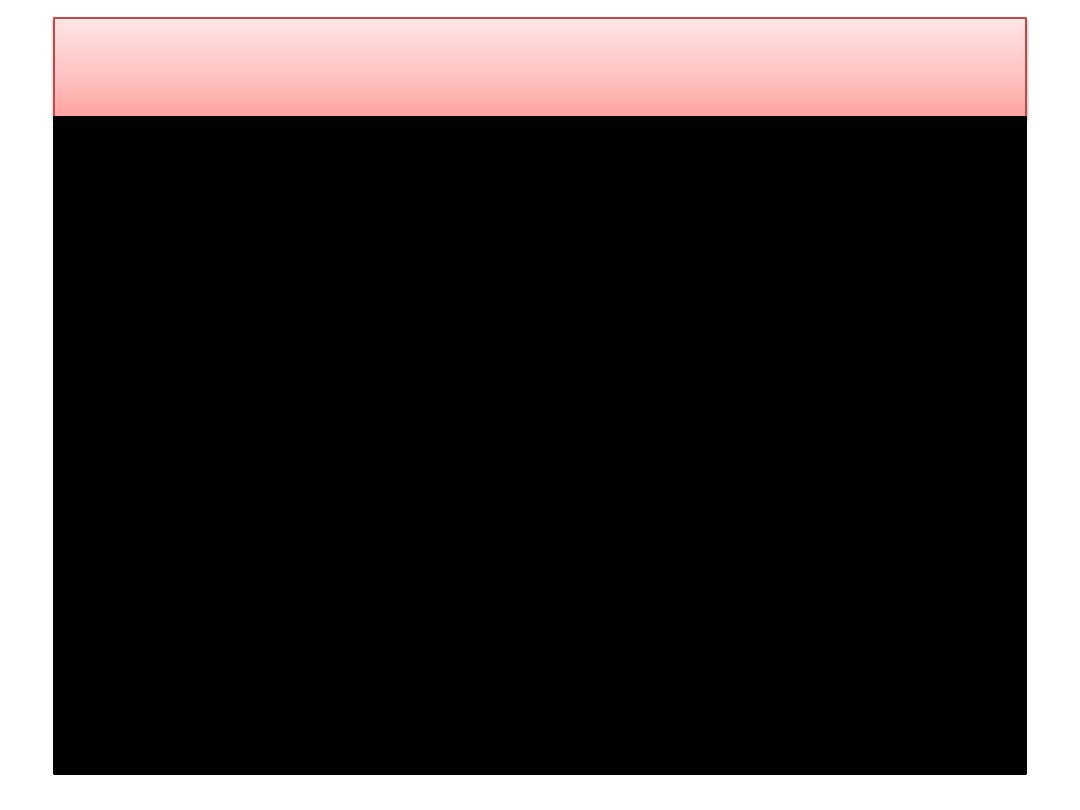
Fate of H
+
in the Urine
• The amount of acid secreted depends upon the subsequent
events in the tubular urine.
• The maximal
H
+
gradient
against which the transport
mechanisms can secrete in humans corresponds to a urine
pH
of about
4.5
, ie, an H
+
concentration in the urine that is
1000
times
the concentration in plasma.
• pH 4.5 is thus the
limiting pH.
• If there were no
buffers
that "
tied up
" H
+
in the urine, this pH
would be reached rapidly, and H
+
secretion would stop.
• However,
three
important reactions in the tubular fluid
remove free H
+
, permitting more acid to be secreted.
• These are the reactions with
HCO
3
-
to form CO
2
and H
2
O, with
HPO
4
2-
to form H
2
PO
4
-
, and with
NH
3
to form NH
4
+
.
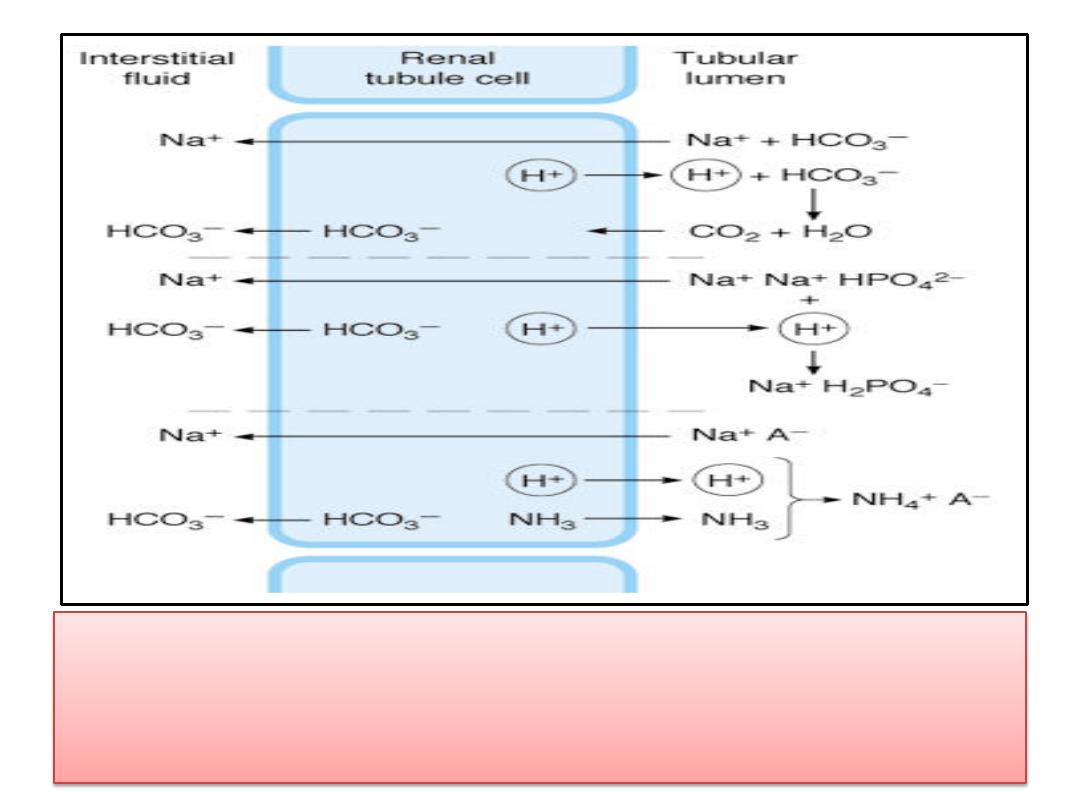
• Fate of H
+
secreted into a tubule in exchange for Na
+
. Top:
Reabsorption of filtered bicarbonate via CO
2
. Middle: Formation of
monobasic phosphate. Bottom: Ammonium formation. Note that in
each instance one Na
+
ion and one HCO
3
-
ion enter the bloodstream
for each H
+
ion secreted. A
-
, anion.
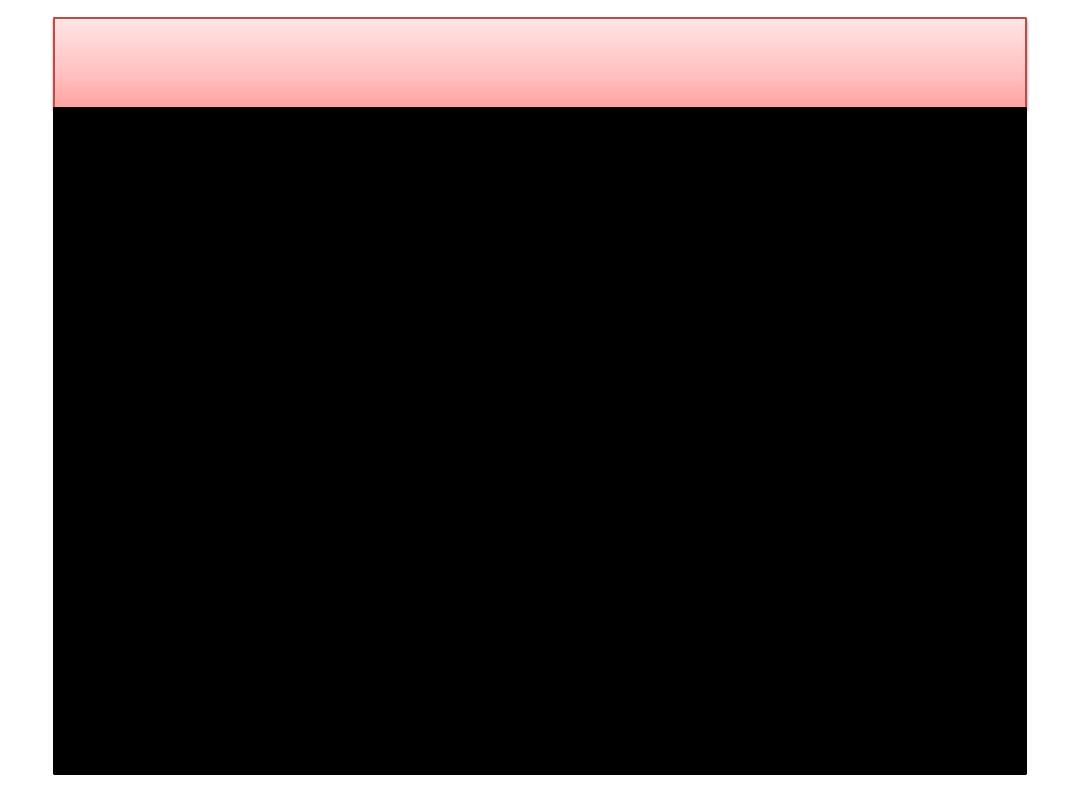
Reaction With Buffers
• In the proximal tubule, most of the secreted H
+
reacts with
HCO
3
-
to form
H
2
CO
3
.
• The
H
2
CO
3
breaks down to form
CO
2
and
H
2
O
.
• In the
proximal
(but not in the distal) tubule, there is
carbonic anhydrase
in the brush border of the cells; this
facilitates the formation of CO
2
and H
2
O in the tubular fluid.
• The CO
2
, which
diffuses readily
across all biological
membranes, enters the tubular cells, where it adds to the
pool of CO
2
available to form H
2
CO
3
.
• Since
most of the H
+
is removed
from the tubule, the pH of
the fluid is changed very little.
• This is the mechanism by which HCO
3
-
is reabsorbed; for
each mole of HCO
3
-
removed from the tubular fluid,
1 mole
of HCO
3
-
diffuses from the tubular cells
into the blood
,
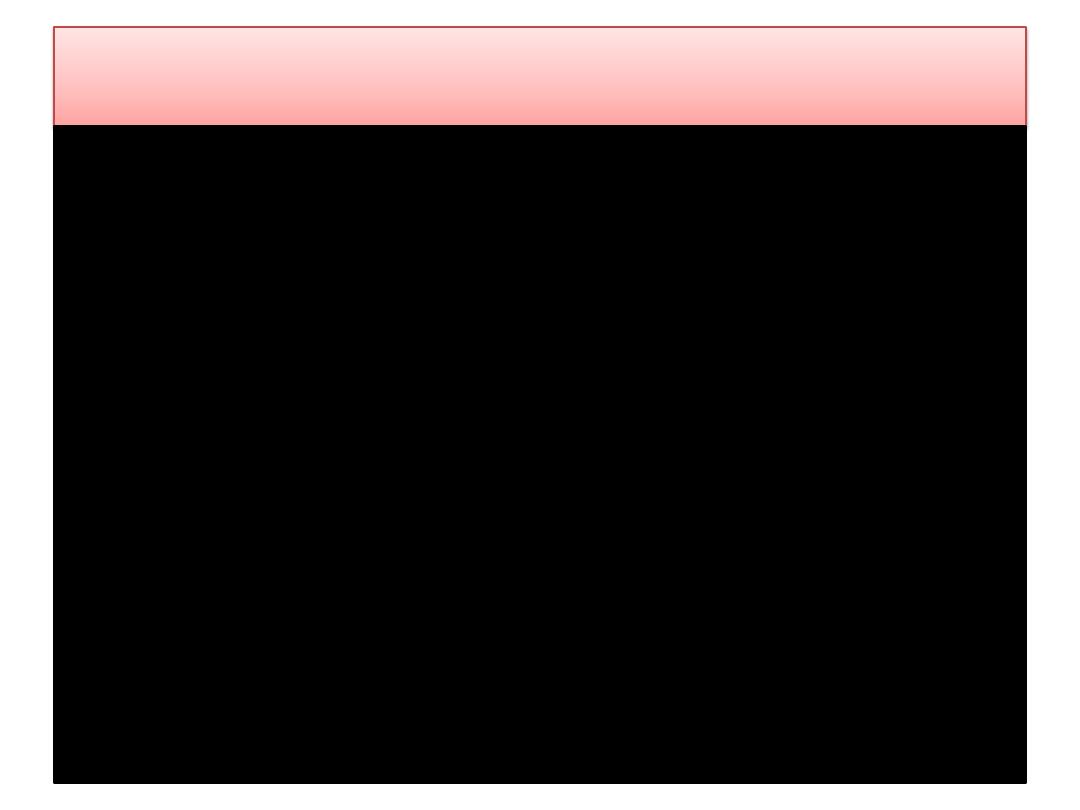
Reaction With Buffers
• Secreted H
+
also reacts with dibasic phosphate
(
HPO
4
2
-
) to form monobasic phosphate
(
H
2
PO
4
-
).
• This happens to the greatest extent in the
distal tubules and collecting
ducts,
• The reaction with
NH
3
occurs in the
proximal
and distal
tubules.
• H
+
also combines to a minor degree with other
buffer
anions.
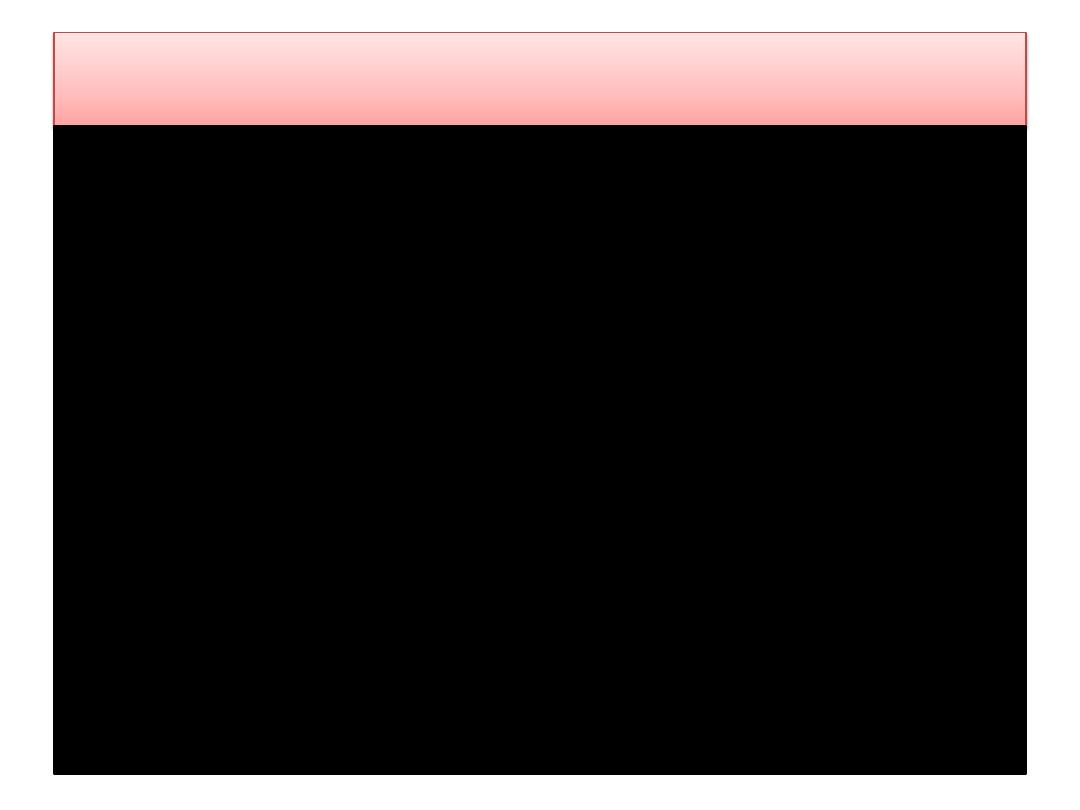
Ammonia Secretion
• Reactions in the renal tubular cells produce
NH
4
+
and
HCO
3
-
.
•
NH
4
+
is in
equilibrium
with
NH
3
+ H
+
in the cells.
• Since the pK´ of this reaction is 9.0, the ratio of
NH
3
to NH
4
+
at pH 7.0 is 1:100 .
• However,
NH
3
is
lipid-soluble
and diffuses across
the cell membranes down its concentration
gradient into the
interstitial fluid
and
tubular
urine
.
• In the urine it reacts with
H
+
to form
NH
4
+
, and
the NH
4
+
remains in the urine.
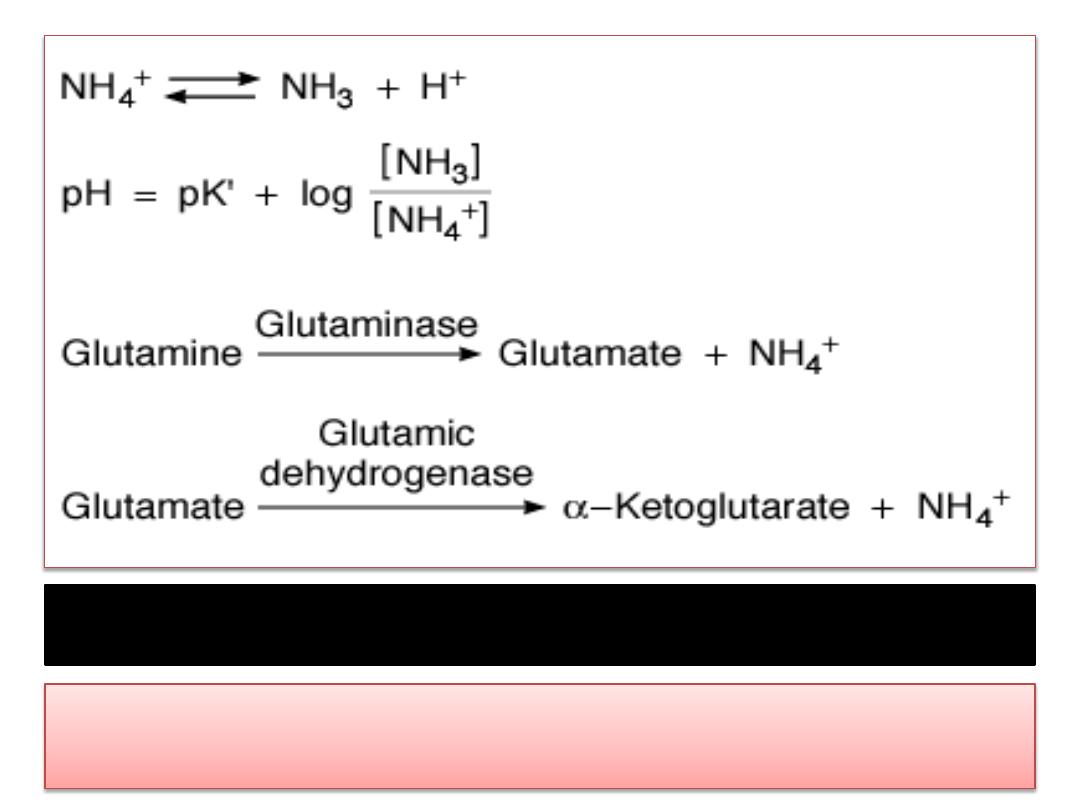
Major reactions involved in ammonia production in the kidneys.
Subsequent metabolism of
α-ketoglutarate utilizes
2H
+
, freeing
2HCO
3
-
.
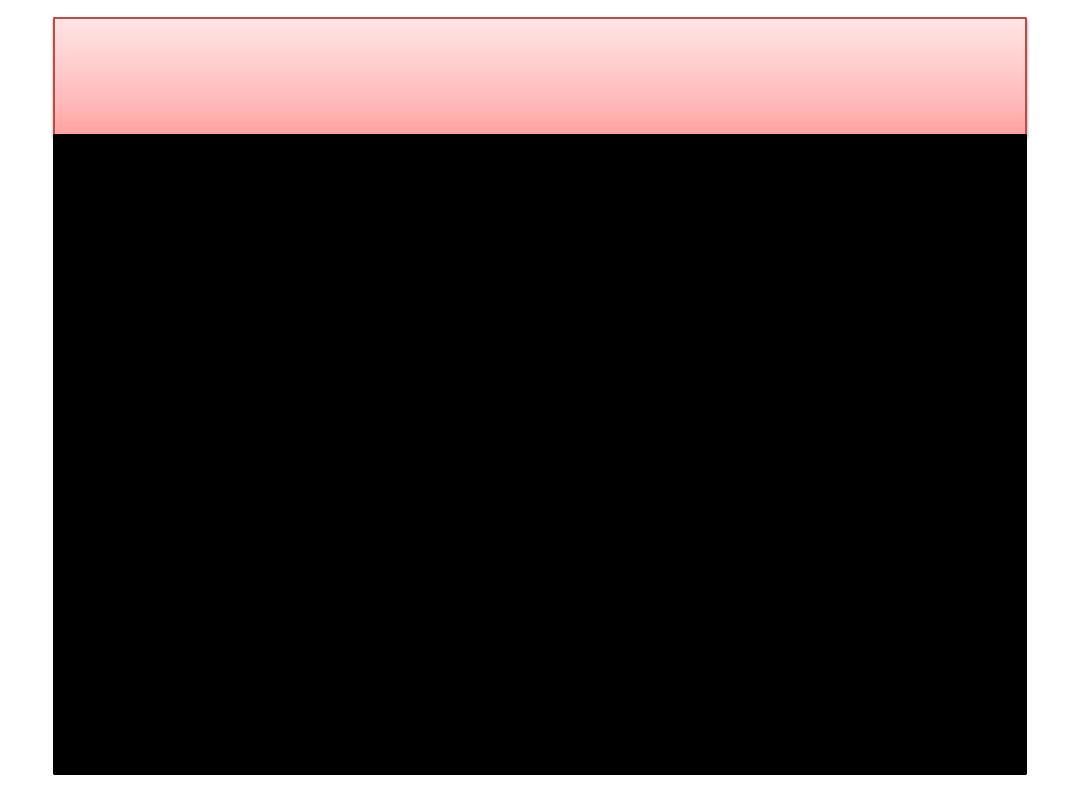
Ammonia Secretion
• The principal reaction producing NH
4
+
in cells is
conversion of
glutamine to glutamate
.
• This reaction is catalyzed by the enzyme
glutaminase,
which is abundant in renal tubular
cells .
• Glutamic dehydrogenase
catalyzes the
conversion of glutamate to
α-ketoglutarate, with
the production of more NH
4
+
.
• Subsequent metabolism of α-ketoglutarate
utilizes
2H
+
, freeing
2HCO
3
-
.
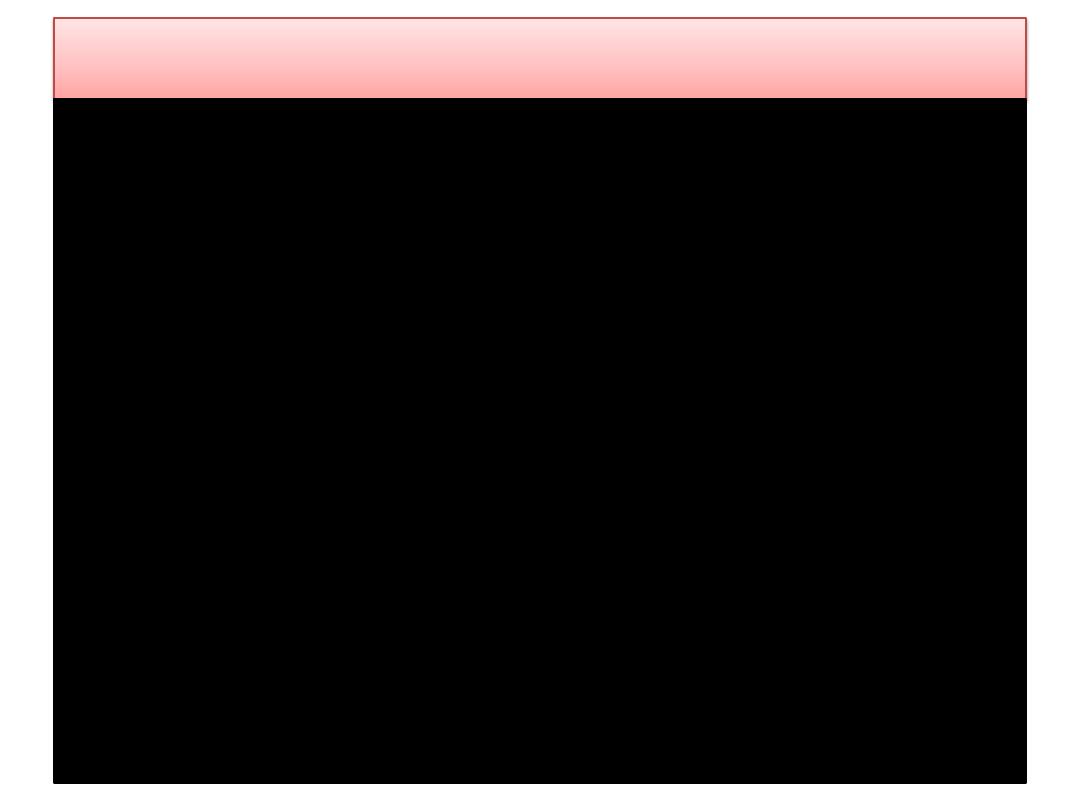
Factors Affecting Acid Secretion
• Renal acid secretion is altered by changes in the
intracellular
PCO
2
,
K
+
concentration, carbonic anhydrase
level, and
adrenocortical
hormone concentration.
• When the PCO
2
is high (respiratory acidosis), more
intracellular H
2
CO
3
is available to buffer the hydroxyl ions and
acid secretion is enhanced, whereas the reverse is true when
the PCO
2
falls.
• K
+
depletion enhances
acid secretion, apparently because the
loss of K
+
causes intracellular acidosis even though the plasma
pH may be elevated.
• Conversely,
K
+
excess
in the cells
inhibits
acid secretion.
• When
carbonic anhydrase is inhibited
, acid secretion is
inhibited, because the formation of H
2
CO
3
is decreased.
• Aldosterone
and the other adrenocortical steroids that
enhance tubular reabsorption of Na
+
also increase the
secretion of H
+
and K
+
.
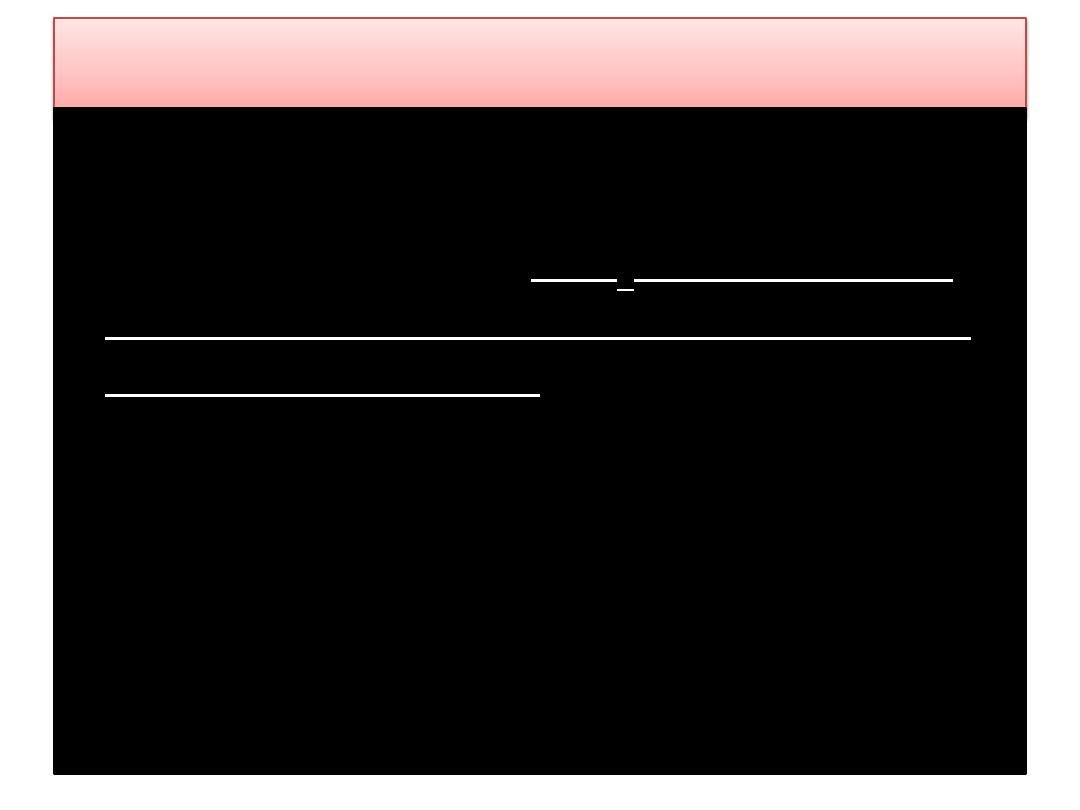
Bicarbonate Excretion
• Although the process of HCO
3
-
reabsorption
does not actually involve
transport of this ion
into the tubular cells, HCO
3
-
reabsorption is
proportionate to the amount filtered over a
relatively wide range.
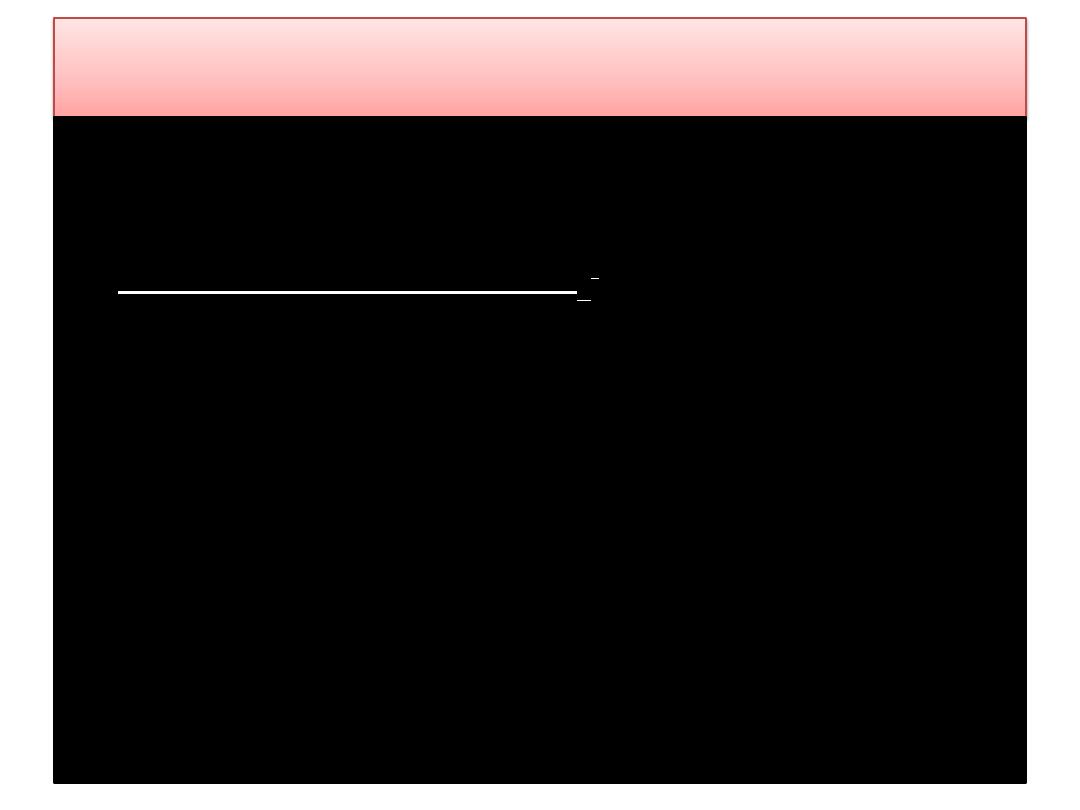
Bicarbonate Excretion
• When the
plasma HCO
3
-
concentration is
low
, all the
filtered HCO
3
-
is
reabsorbed
; but when the plasma
HCO
3
-
concentration is
high
, ie, above 26-28 meq/L
(the renal threshold for HCO
3
-
),
HCO
3
-
appears
in the
urine
and the urine becomes alkaline.
• Conversely, when the plasma HCO
3
-
falls
below about
26 meq/L, the value at which all the secreted H
+
is
being used to reabsorb HCO
3
-
, more H
+
becomes
available to combine with other buffer anions.
• Therefore, the
lower
the plasma
HCO
3
-
concentration
drops, the more
acidic the urine
becomes and the
greater its
NH
4
+
content.
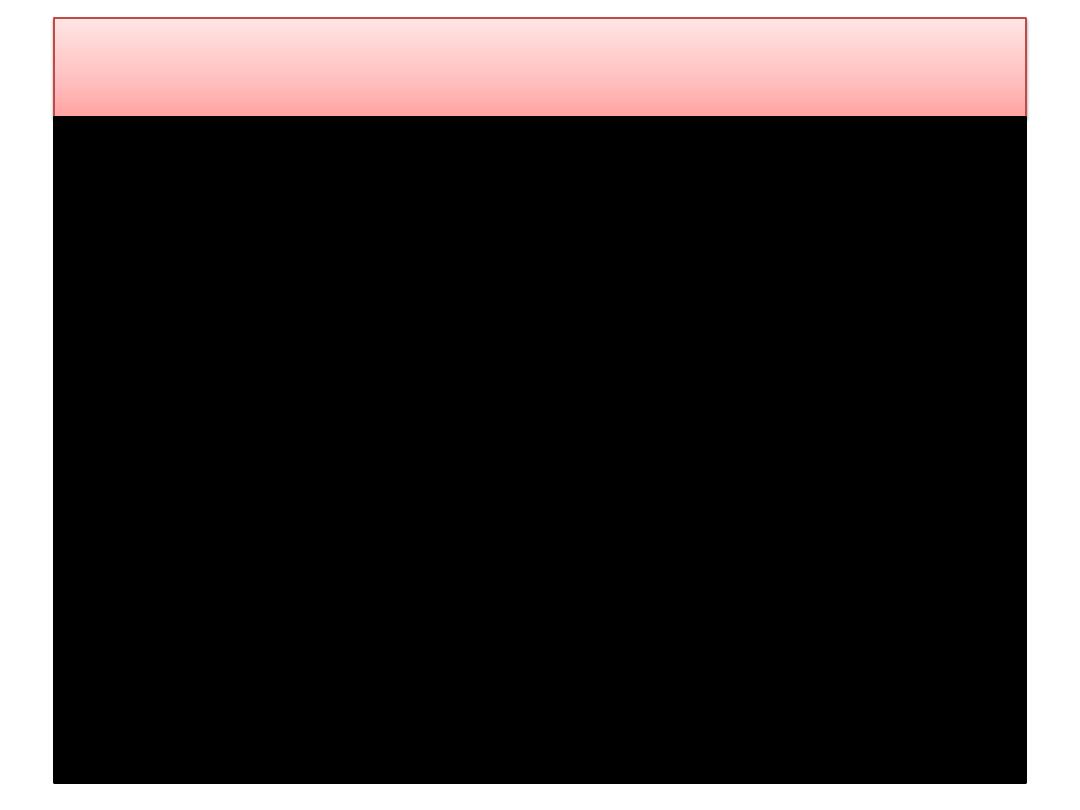
Implications of Urinary pH Changes
• Depending upon the rates of the
interrelated processes
of acid secretion, NH
4
+
production, and HCO
3
-
excretion, the pH of the urine in humans varies from
4.5 to 8.0.
• Acids are buffered in the plasma and cells, the overall
reaction being HA + NaHCO
3
→ NaA + H
2
CO
3
.
• The H
2
CO
3
forms CO
2
and H
2
O, and the CO
2
is expired,
while the NaA appears in the glomerular filtrate.
• To the extent that the Na
+
is replaced by H
+
in the
urine, Na
+
is conserved in the body.
• Furthermore, for
each H
+
ion
excreted with phosphate
or as NH
4
+
, there is a net gain of
one HCO
3
-
ion in the
blood, replenishing the supply of this important buffer
anion.
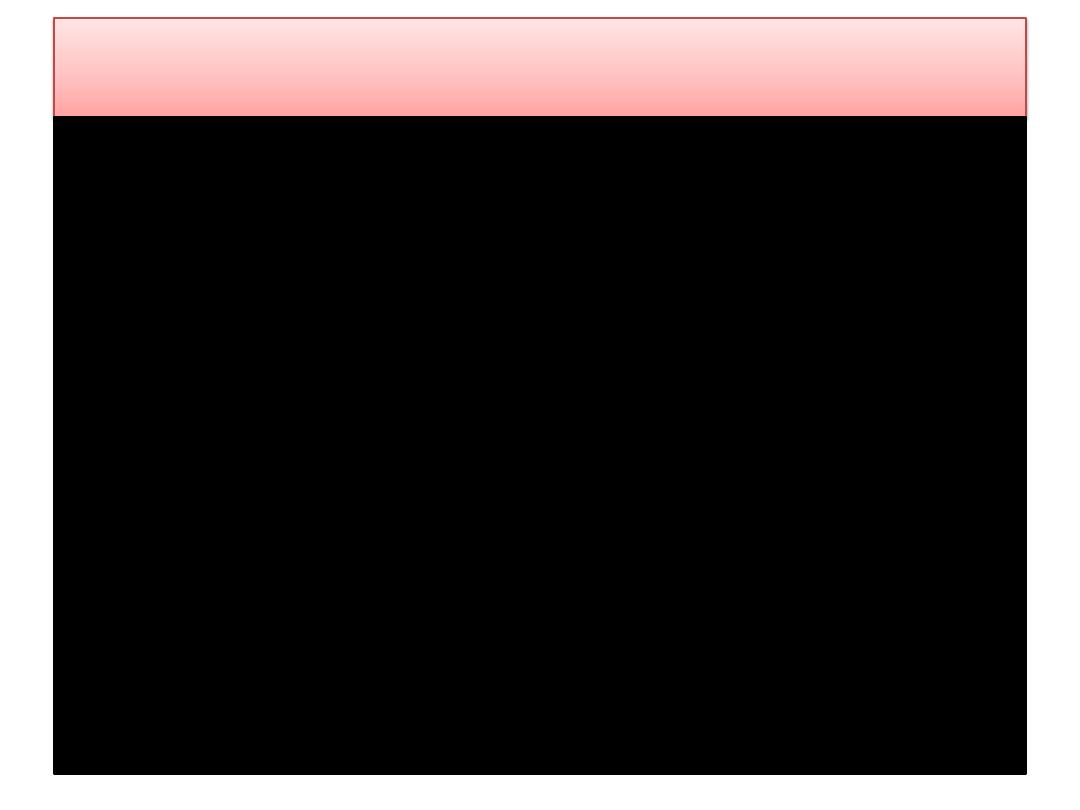
Implications of Urinary pH Changes
• Conversely, when base is added to the body
fluids,
the OH
-
ions are buffered, raising the
plasma HCO
3
-
.
• When the plasma level exceeds
28 meq/L
, the
urine becomes alkaline and the extra HCO
3
-
is
excreted in the urine.
• Because the rate of maximal H
+
secretion by
the tubules varies directly with the arterial
PCO
2
, HCO
3
-
reabsorption also is affected by
the PCO
2
.
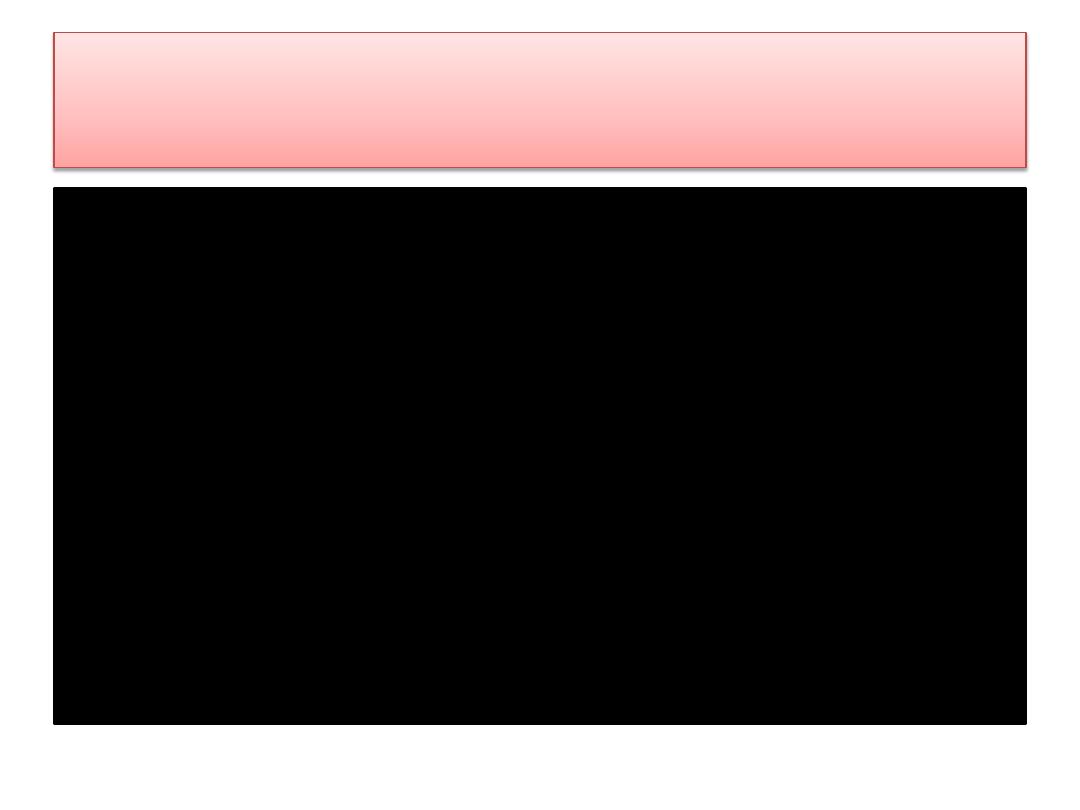
بسم ه الرحمن الرحيم
Regulation of Extracellular
Fluid Composition & Volume
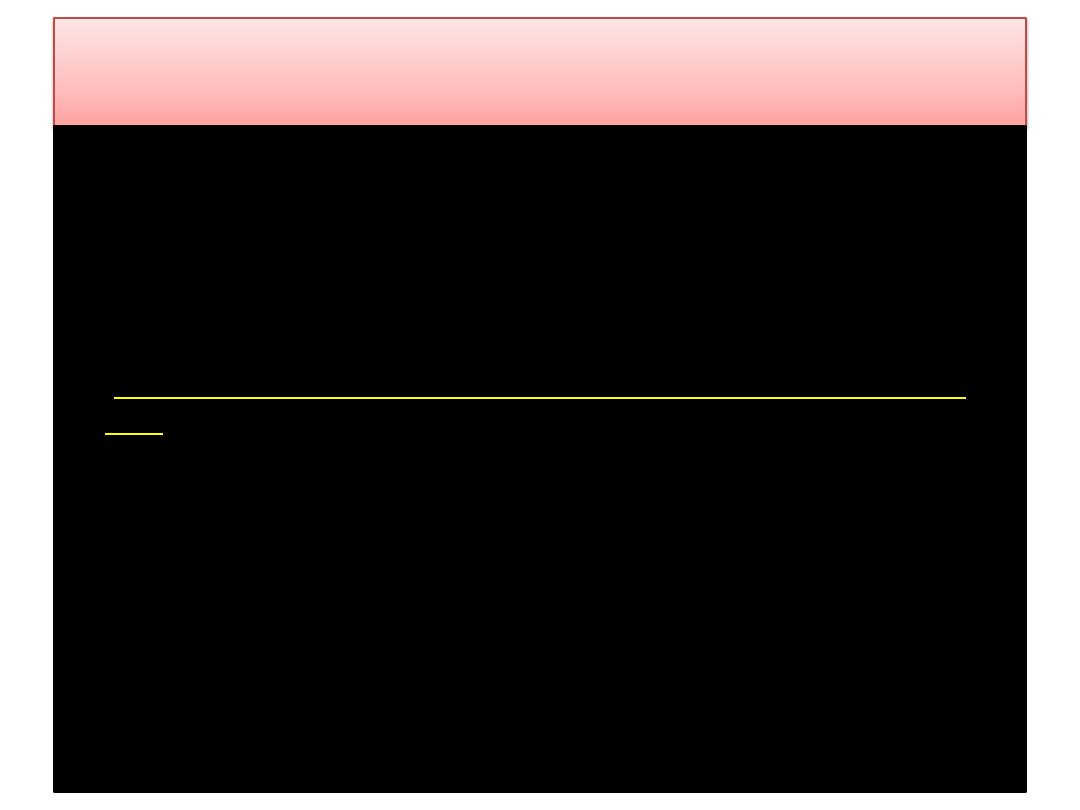
DEFENSE OF H
+
CONCENTRATION
• The
pH notation
is a useful means of expressing
H
+
concentrations
in the body, because the H
+
concentrations
happen to be low relative to those of other cations.
• Thus, the normal Na
+
concentration of arterial plasma that
has been equilibrated with red blood cells is about
140
meq/L
, whereas the H
+
concentration is
0.00004 meq/L
.
•
The pH, the negative logarithm of 0.00004, is therefore
7.4.
• Of course, a decrease in
pH of 1 unit
, eg, from 7.0 to 6.0,
represents
a tenfold increase
in H
+
concentration.
• It is important to remember that the pH of blood is the pH
of true plasma
—plasma that has been in equilibrium with
red cells
—because the red cells contain hemoglobin, which
is quantitatively one of the most important blood buffers .
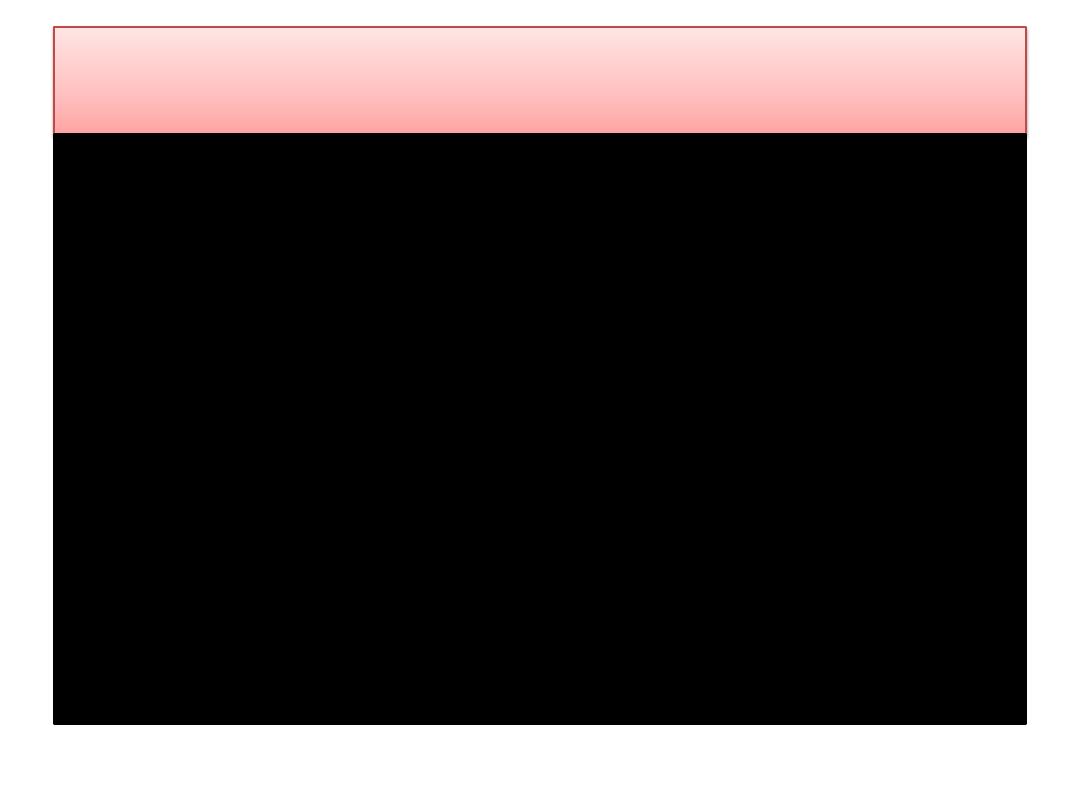
H
+
Balance
• The pH of the arterial plasma is normally
7.40
and that of venous plasma slightly
lower.
• Technically,
acidosis
is present whenever
the arterial pH is below
7.40
, and
alkalosis
is present whenever it is above
7.40
, although variations of up to
0.05
pH
unit occur without untoward effects.
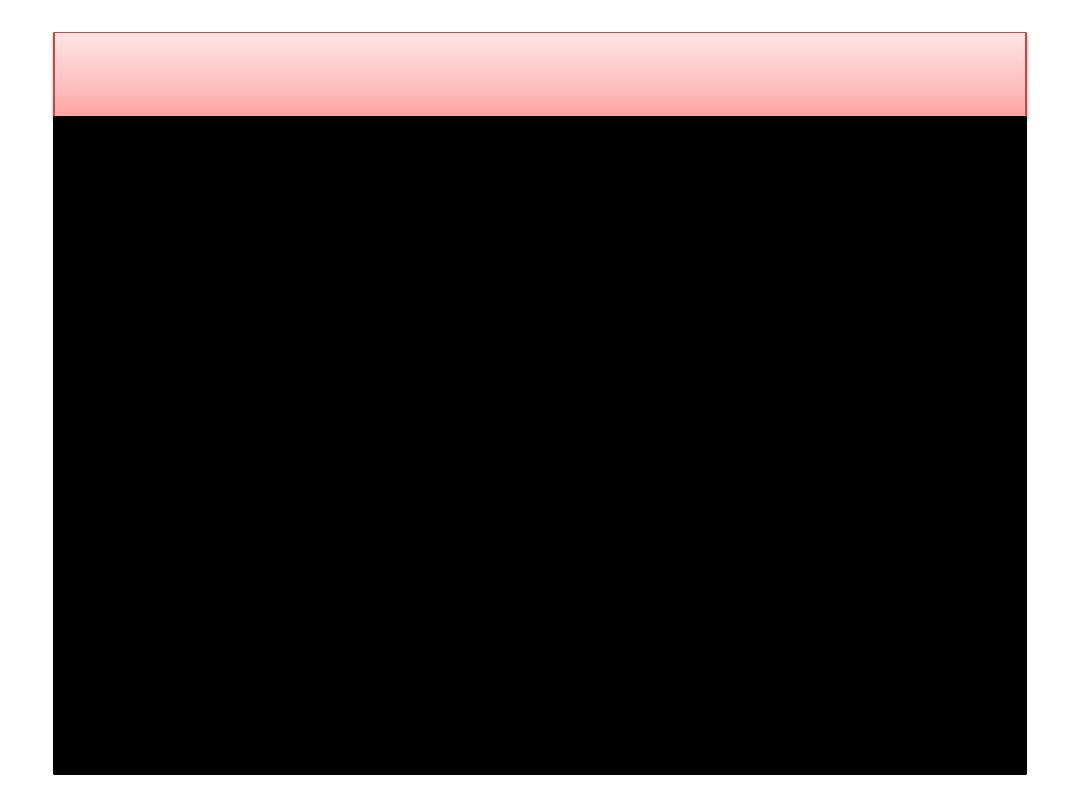
H
+
Balance
• Amino acids are utilized in the liver for
gluconeogenesis
, leaving as products
NH
4
+
and
HCO
3
-
from their amino and carboxyl groups .
• The
NH
4
+
is incorporated into
urea
and the
protons
that are formed are buffered
intracellularly by
HCO
3
-
, so little NH
4
+
and HCO
3
-
escape into the circulation.
• However, metabolism of
sulfur-containing
amino
acids produces
H
2
SO
4
, and metabolism of
phosphorylated
amino acids such as
phosphoserine produces
H
3
PO
4
.
• These
strong acids
enter the circulation and
present a major H
+
load to the buffers in the ECF.
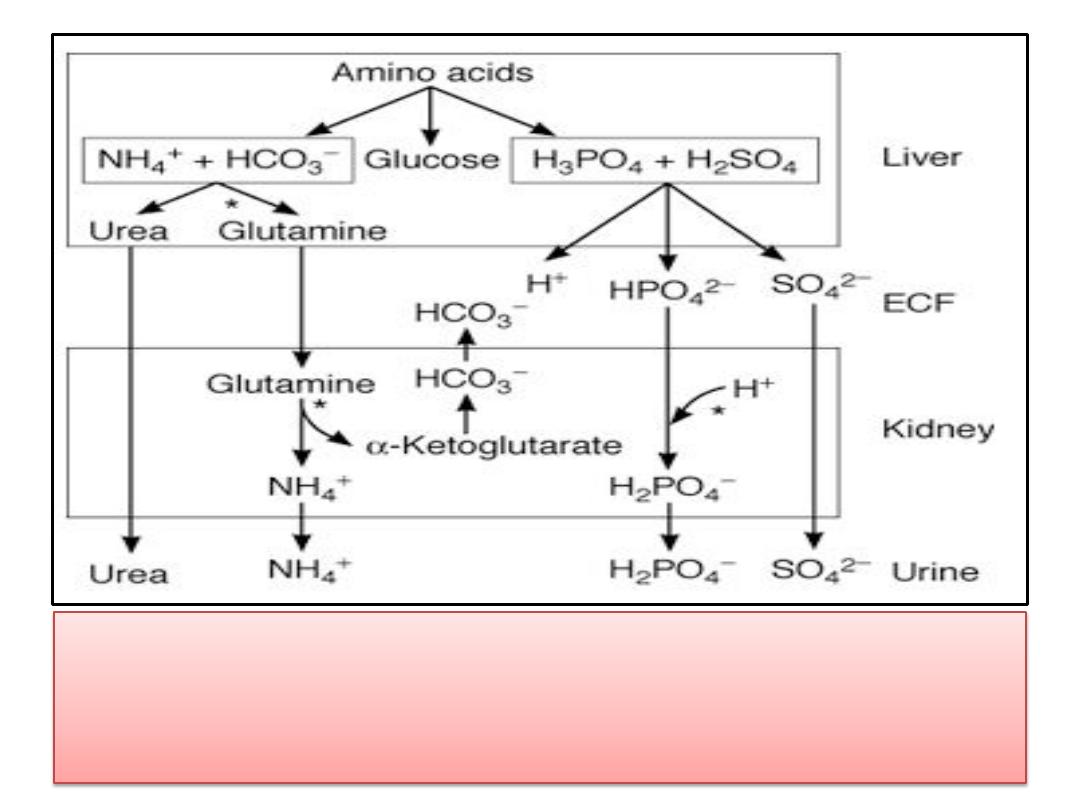
• Role of the liver and kidneys in the handling of
metabolically produced acid loads. Sites where
regulation occurs are indicated by asterisks.
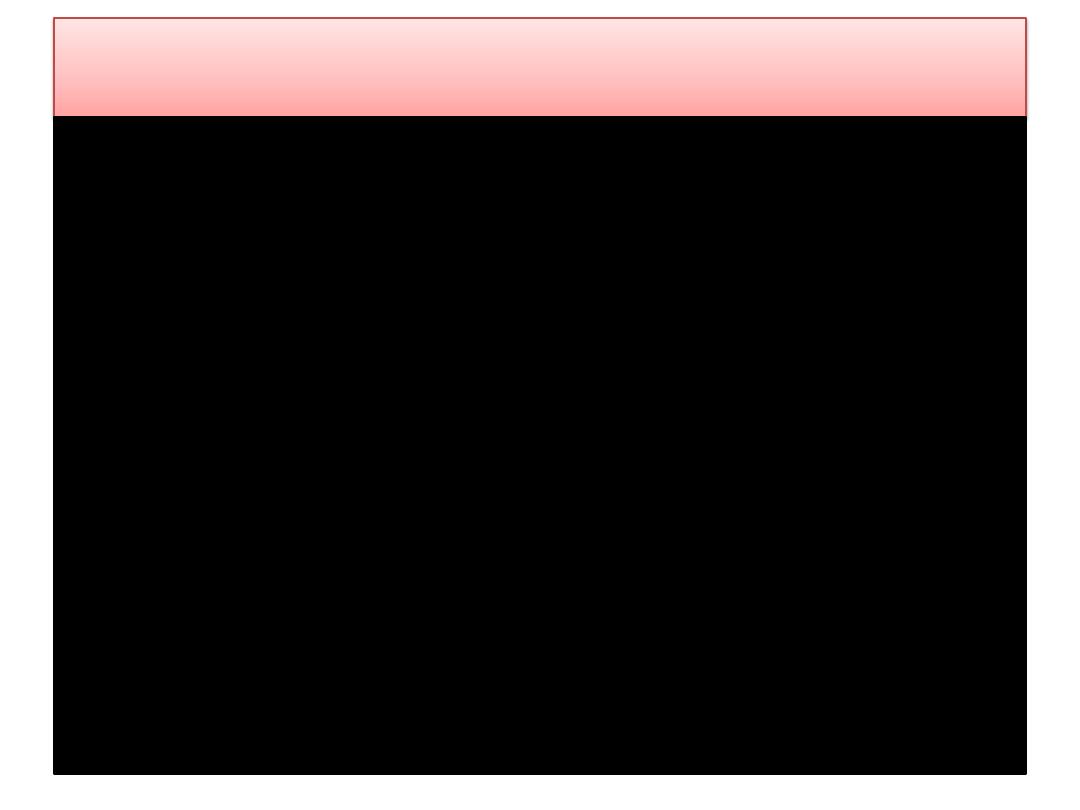
H
+
Balance
• The H
+
load from
amino acid
metabolism is
normally about
50 meq/d
.
• The
CO
2
formed by metabolism in the tissues
is in large part
hydrated to H
2
CO
3
, and the
total H
+
load from this source is over
12,500
meq/d.
• However, most of the
CO
2
is excreted in the
lungs, and only small quantities of the H
+
remain to be excreted by the kidneys.
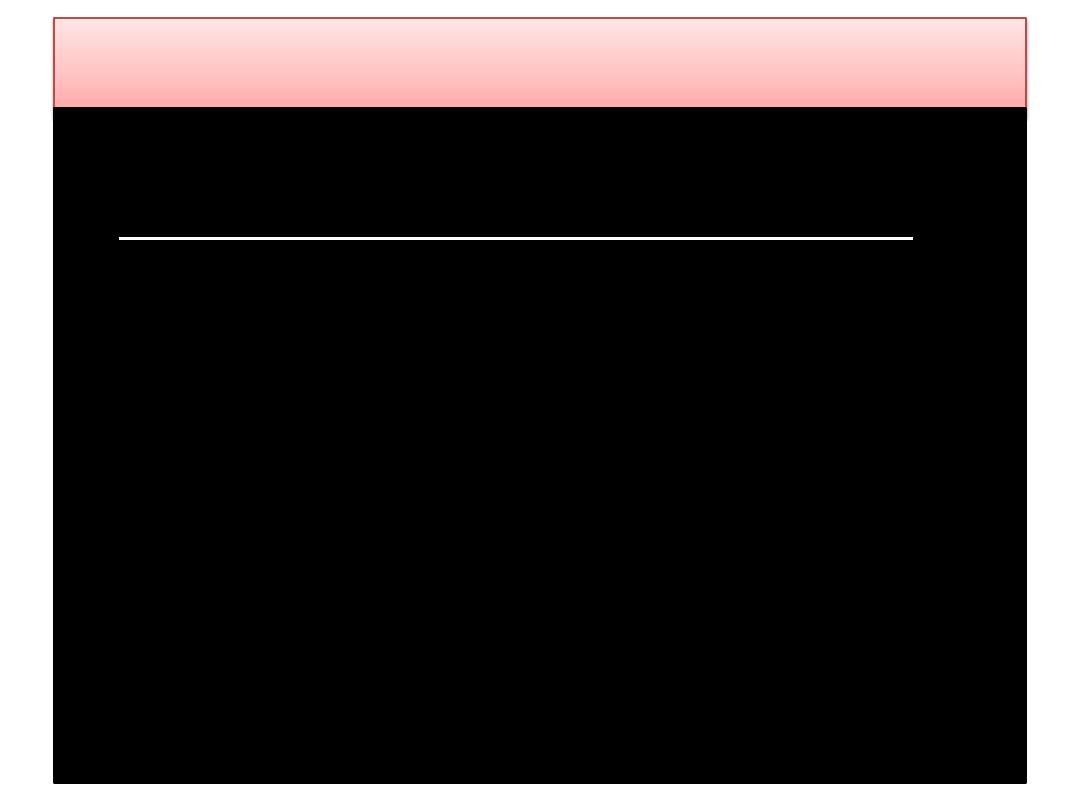
H
+
Balance
• Common sources of extra acid loads are
strenuous exercise (
lactic acid
),
diabetic ketosis
(acetoacetic
acid and β-hydroxybutyric acid),
and
ingestion
of acidifying salts such as
NH
4
Cl
and
CaCl
2
, which in effect add HCl to the body.
• Failure of diseased kidneys to excrete normal
amounts of acid is also a cause of acidosis.
• Fruits
are the main dietary source of alkali.
• but a more common cause of alkalosis is
loss of
acid
from the body as a result of
vomiting
of
gastric juice rich in HCl. This is, of course,
equivalent to adding alkali to the body.
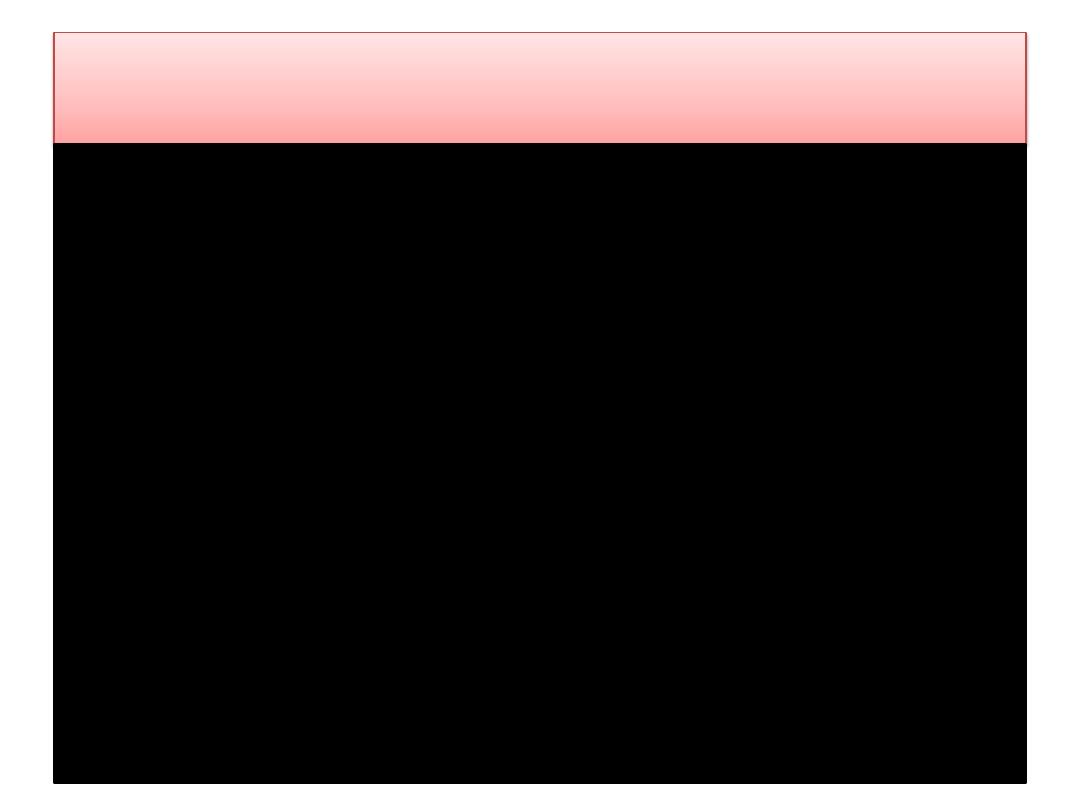
Buffering
• Intracellular and extracellular pH are generally
maintained at very constant levels.
• For example, the pH of the ECF is
7.40
, and in
health, this value usually varies less than
±0.05
pH unit.
• Body pH is stabilized by the
buffering capacity
of
the body fluids.
• A buffer is a substance that has the ability to
bind
or release H
+
in solution, thus keeping the pH of
the solution relatively constant despite the
addition of considerable quantities of
acid or
base
.
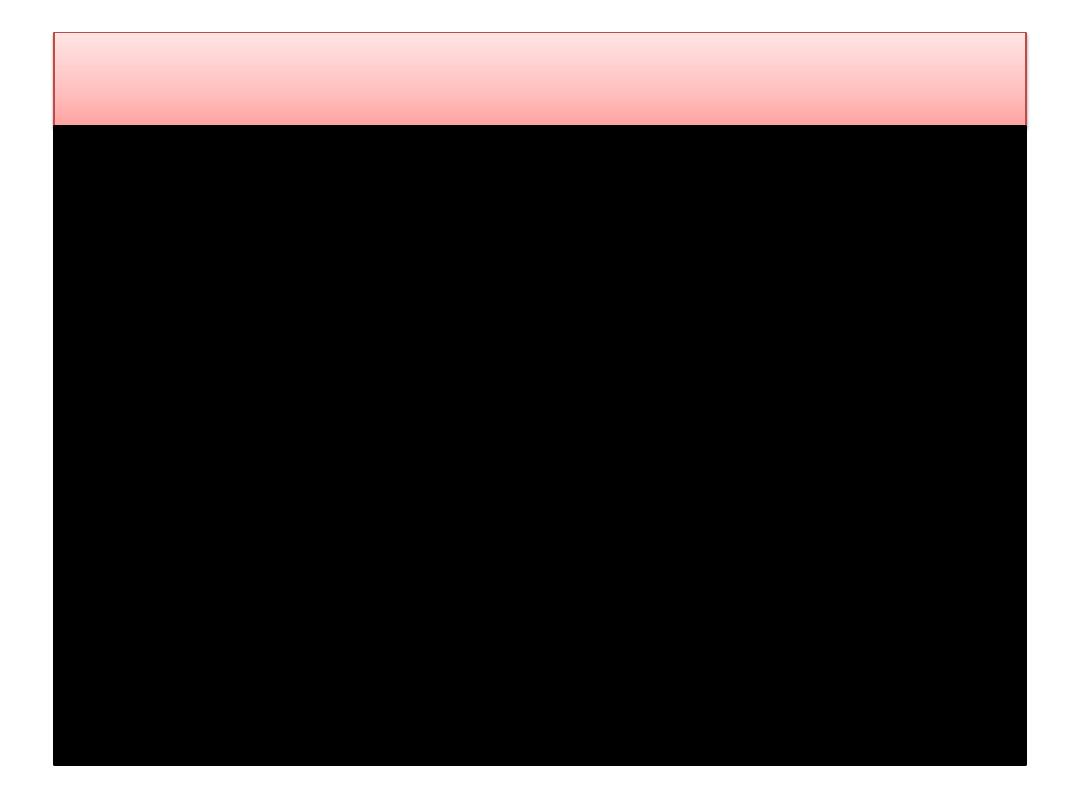
Buffering
• One buffer in the body is
carbonic acid
. This acid
is only
partly dissociated
into H
+
and bicarbonate:
H
2
CO
3
↔→ H
+
+ HCO
3
-
.
• If H
+
is added to a solution of carbonic acid, the
equilibrium
shifts to the left
and most of the
added H
+
is removed from solution.
• If
OH
-
is added, H
+
and OH
-
combine, taking H
+
out of solution. However, the decrease is
countered by more dissociation of H
2
CO
3
, and the
decline in H
+
concentration is minimized.
• Other buffers include the
blood proteins
and the
proteins
in cells .
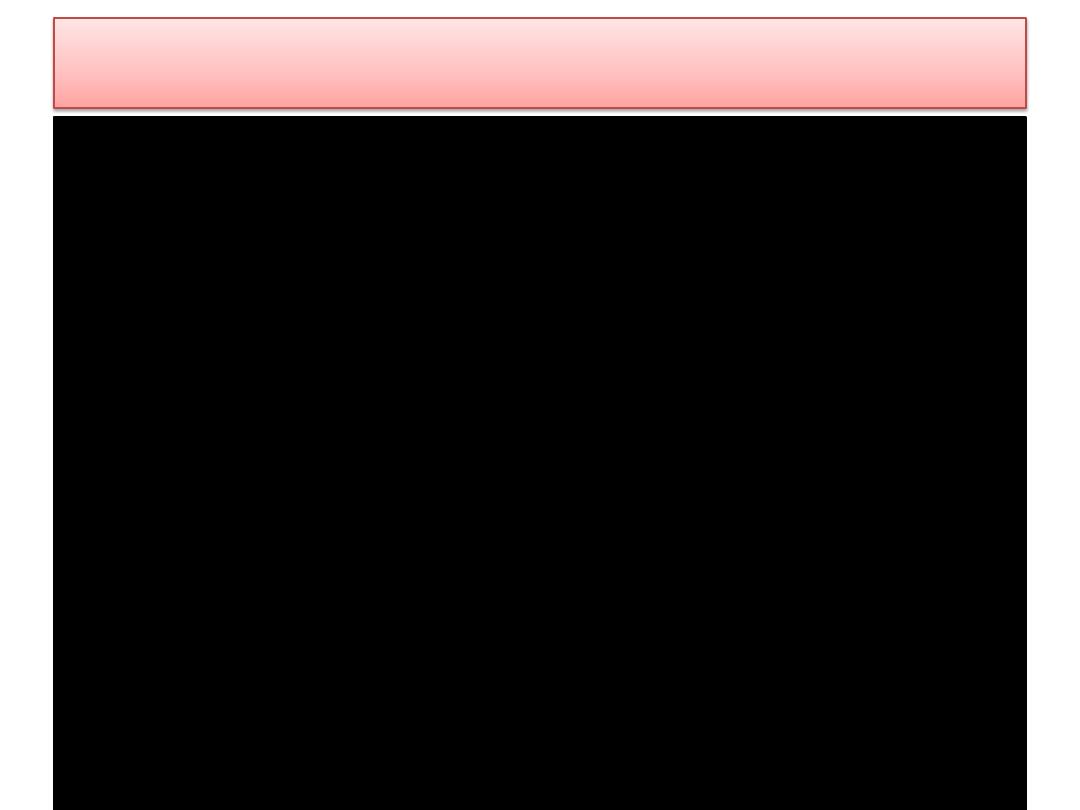
The Henderson-Hasselbalch Equation
• The general equation for a buffer system is
• HA ↔ H+ + A-
A
-
represents any
anion
and
HA
the undissociated
acid.
• If an acid stronger than HA is added to a solution
containing this system, the equilibrium is
shifted to
the left
.
• Hydrogen ions are "
tied up
" in the formation of more
undissociated HA, so the increase in
H
+
concentration
is much less than it would otherwise be.
• Conversely
, if a base is added to the solution, H
+
and
OH
-
react to form H
2
O; but more HA dissociates,
limiting the decrease in H
+
concentration.
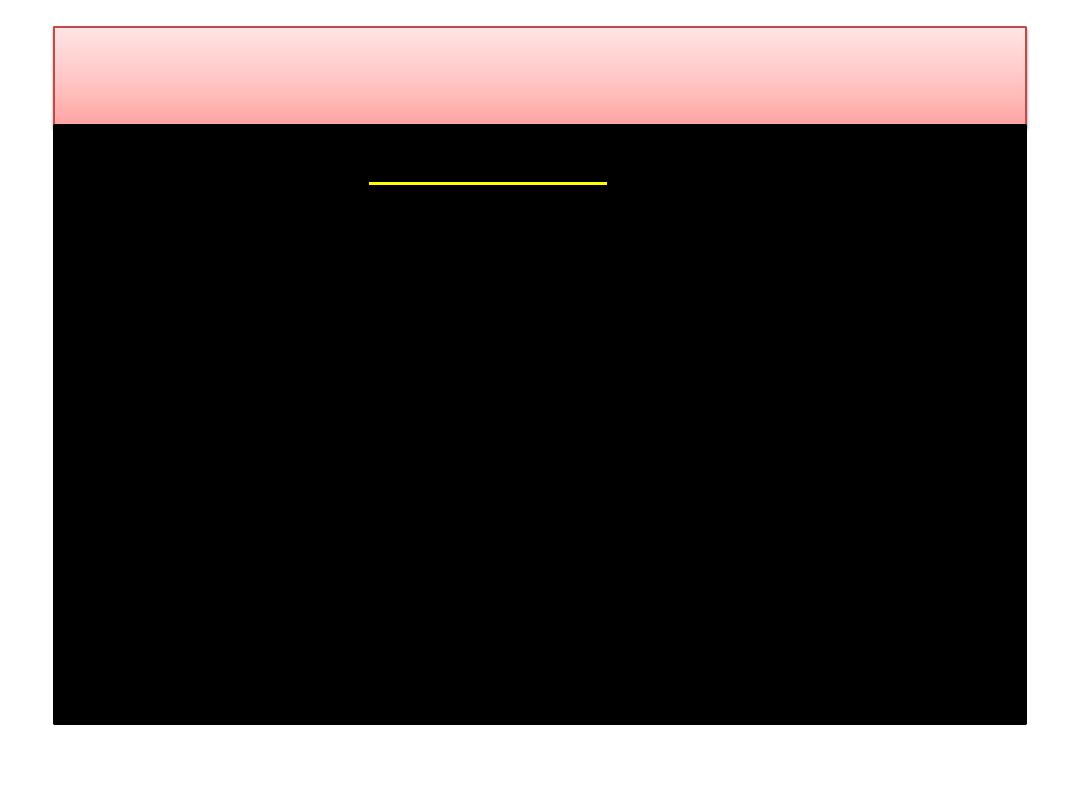
The Henderson-Hasselbalch Equation
• By the law of
mass action
, the product of the
concentrations of the products in a chemical
reaction divided by the product of the
concentration of the reactants at equilibrium
is a constant:
[H] [A-]/[HA] = K
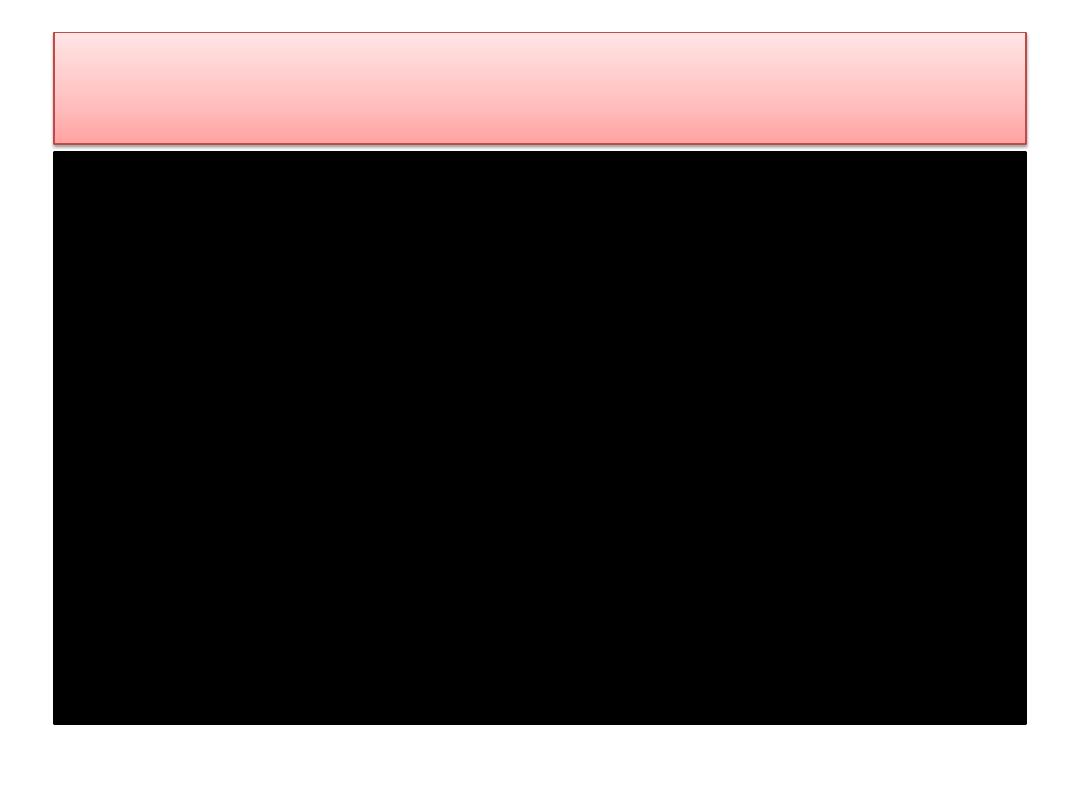
The Henderson-Hasselbalch Equation
• If this equation is solved for H
+
and put in pH
notation (
pH is the negative log of [H
+
]
), the
resulting equation is that originally derived by
Henderson and Hasselbalch to describe the pH
changes resulting from addition of H
+
or OH
-
to any buffer system (Henderson-Hasselbalch
equation):
PH = PK + log [A-] / [AH]
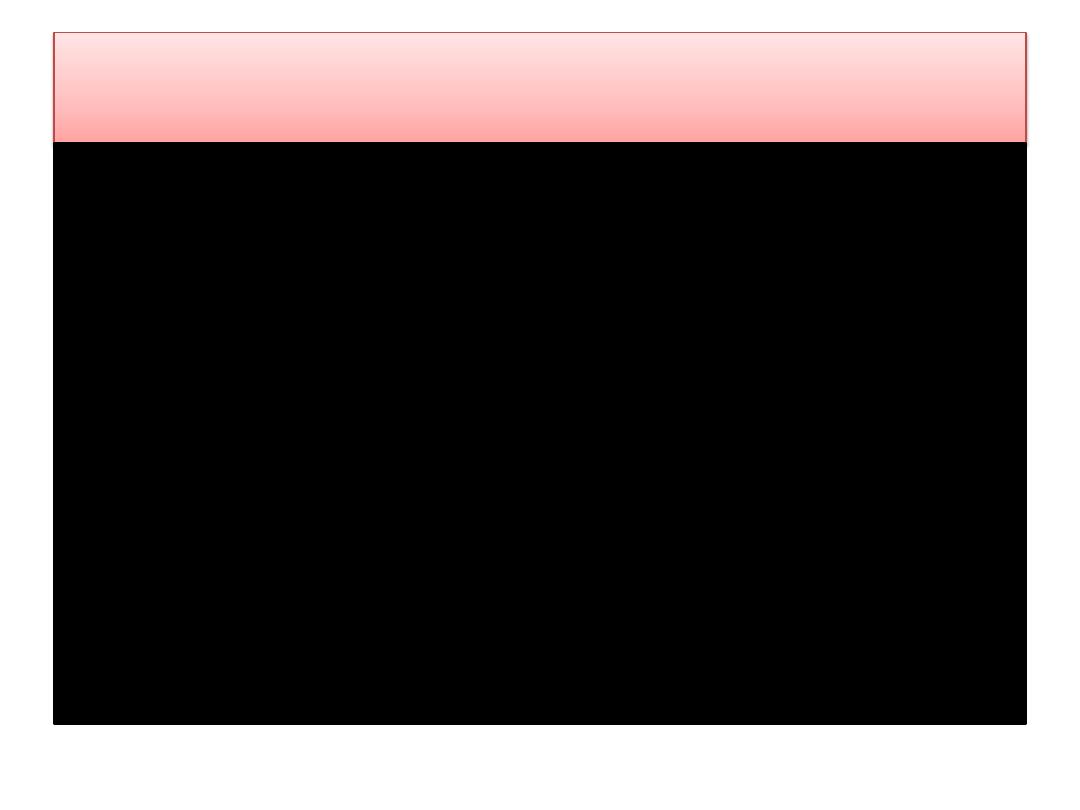
The Henderson-Hasselbalch Equation
• It is apparent from these equations that the buffering capacity
of a system is greatest when the amount of
free anion
is equal
to the amount of
undissociated HA
, ie, when [A
-
]/[HA] = 1, so
that log [A
-
]/[HA] = 0 and pH = pK.
• This is why the most effective buffers in the body would be
expected to be those with
pKs close to the pH
in which they
operate.
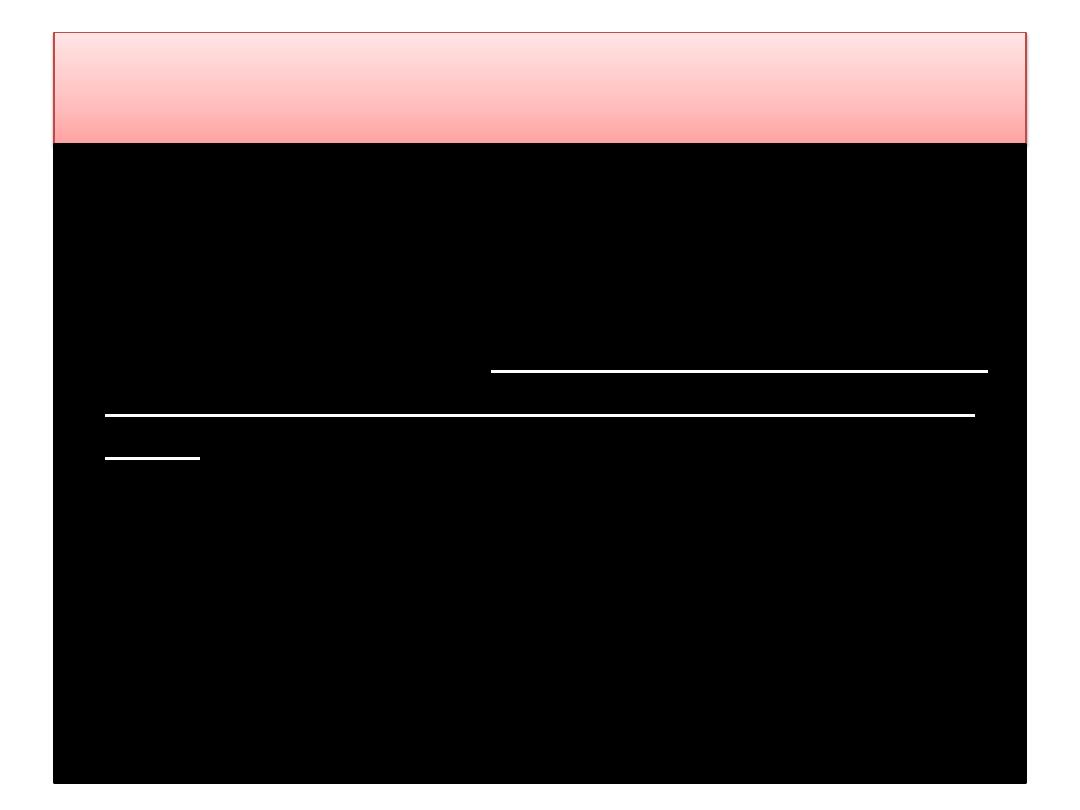
DEFENSE OF TONICITY
• The defense of the tonicity of the ECF is primarily
the function of the
vasopressin
-secreting and
thirst
mechanisms.
• The total body
osmolality
is directly
proportionate to the total body sodium plus the
total body potassium divided by the total body
water, so that changes in the osmolality of the
body fluids occur when there is a disproportion
between the amount of these
electrolytes
and
the amount of
water
ingested or lost from the
body .
• When the effective
osmotic pressure
of the
plasma rises,
vasopressin
secretion is increased
and the
thirst
mechanism is stimulated.
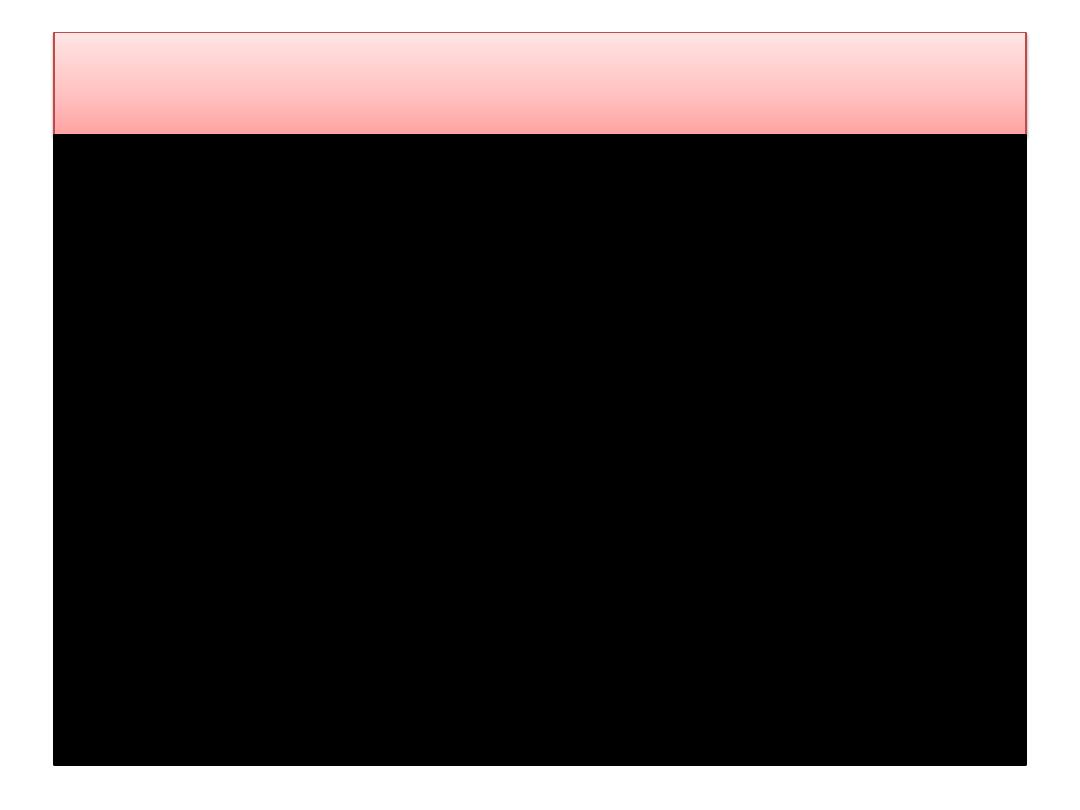
DEFENSE OF TONICITY
• Conversely, when the plasma becomes
hypotonic, vasopressin secretion is decreased
and "solute-free water" (
water in excess of
solute
) is excreted.
• In this way, the tonicity of the body fluids is
maintained within a narrow normal range.
• In health, plasma osmolality ranges from
280
to 295
mosm/kg of H
2
O, with vasopressin
secretion maximally inhibited at 285 mosm/kg
and stimulated at higher values .
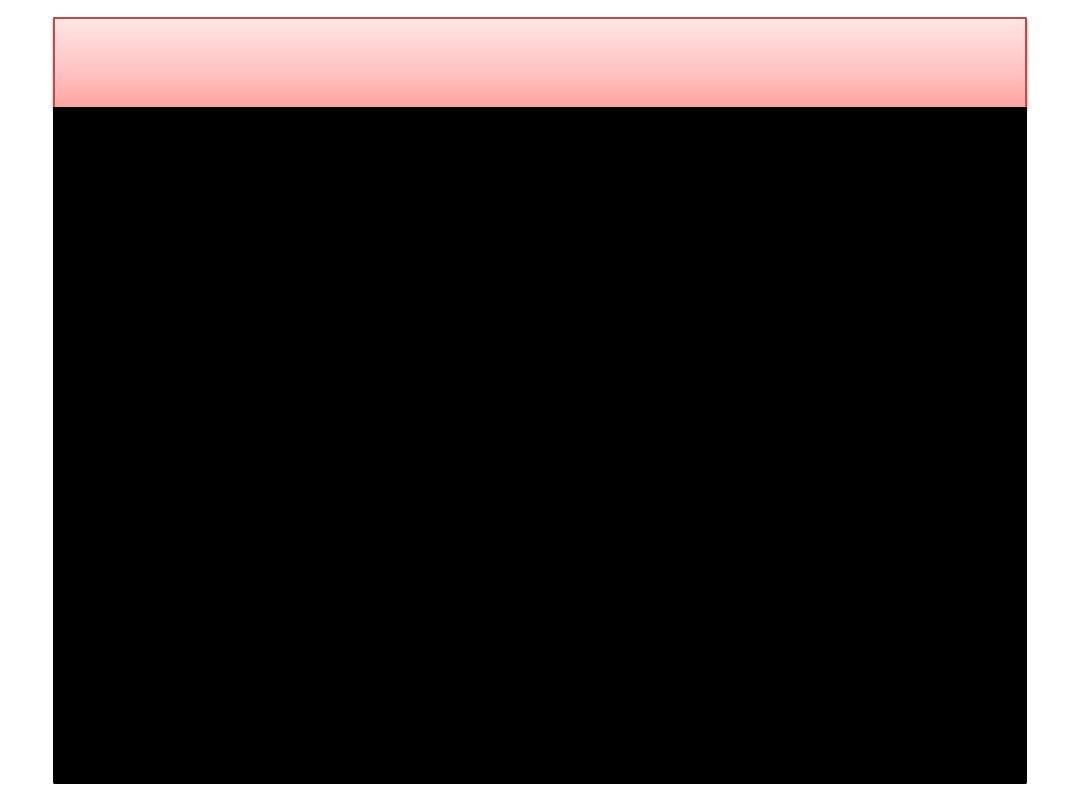
DEFENSE OF VOLUME
• The volume of the
ECF
is determined primarily by the
total amount of osmotically active solute in the ECF.
• Since
Na
+
and Cl
-
are by far the
most abundant
osmotically active solutes in ECF, and
since changes in
Cl
-
are to a great extent secondary to changes in Na
+
,
the amount of Na
+
in the ECF is the most important
determinant of ECF volume.
• Therefore, the mechanisms that control
Na
+
balance
are the major mechanisms defending ECF volume.
• There is, however, a
volume control
of
water excretion
as well; a rise in ECF volume inhibits vasopressin
secretion, and a decline in ECF volume produces an
increase in the secretion of this hormone.
•
Volume stimuli
override the
osmotic regulation
of
vasopressin secretion.
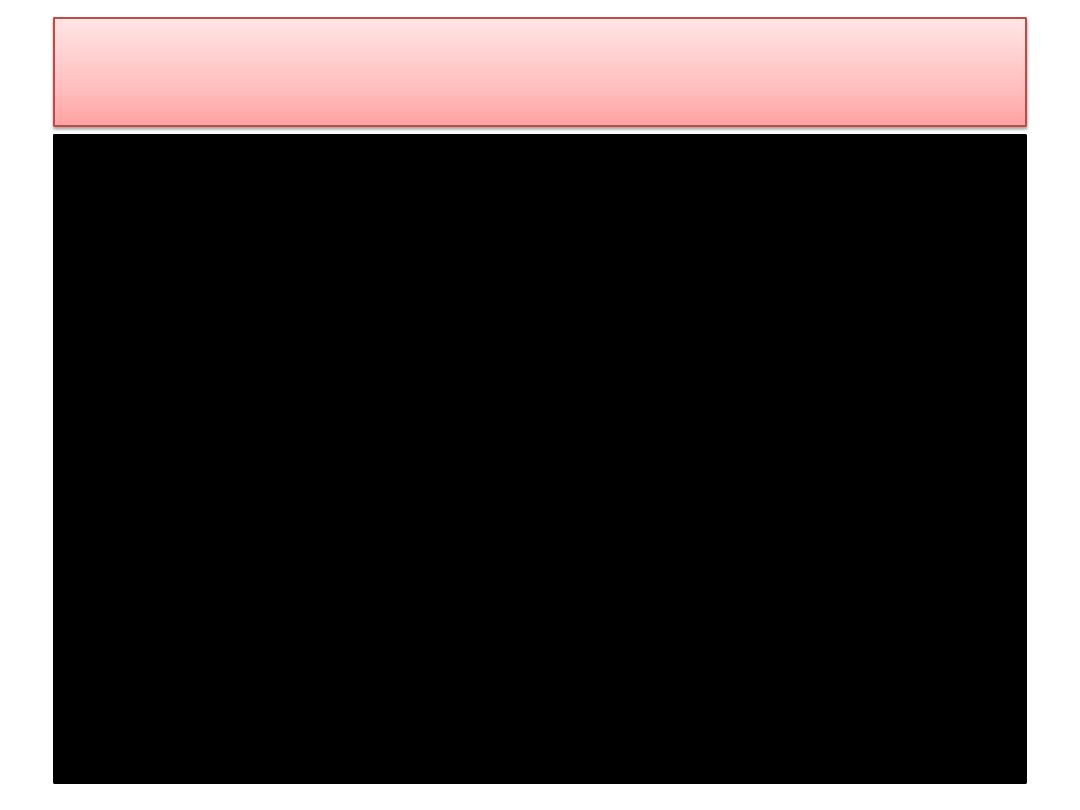
DEFENSE OF VOLUME
•
Angiotensin II
stimulates aldosterone and
vasopressin secretion.
• It also causes
thirst and constricts
blood
vessels, which help to maintain blood
pressure.
• Thus,
angiotensin II
plays a key role in the
body's response to hypovolemia .
• In addition, expansion of the ECF volume
increases the secretion of
ANP
and
BNP
by the
heart, and this causes
natriuresis
and
diuresis
.
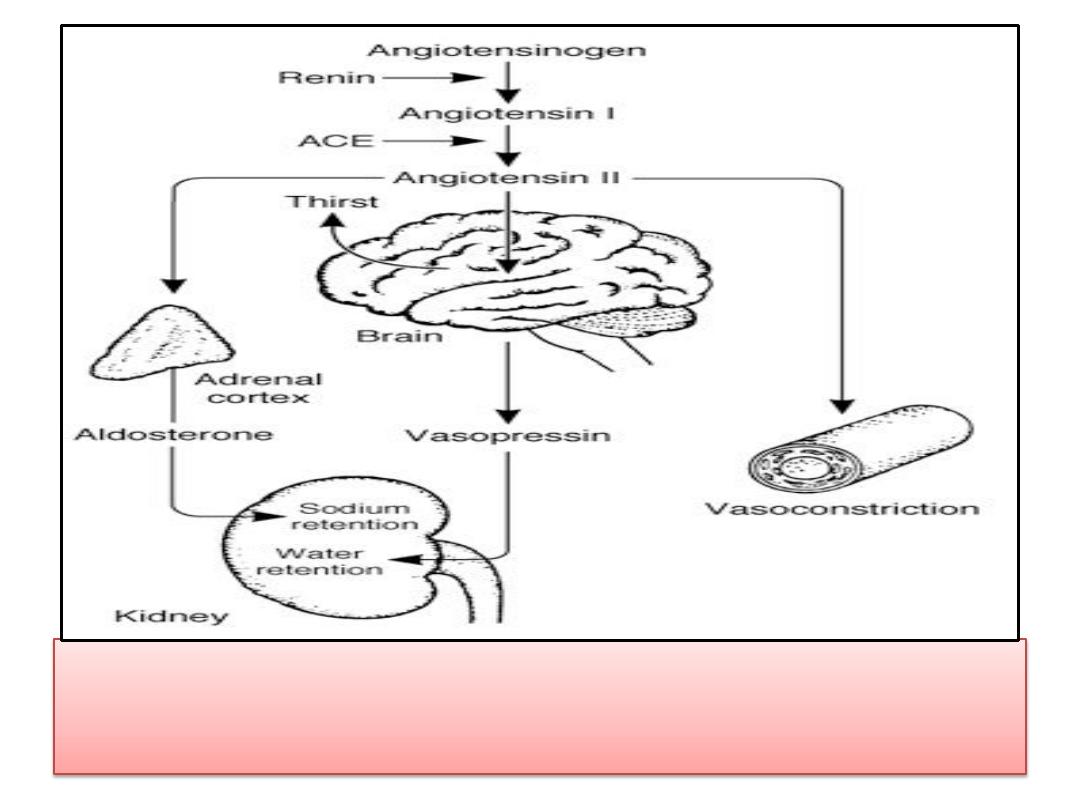
• Defense of ECF volume by angiotensin II. ACE,
angiotensin-converting enzyme.