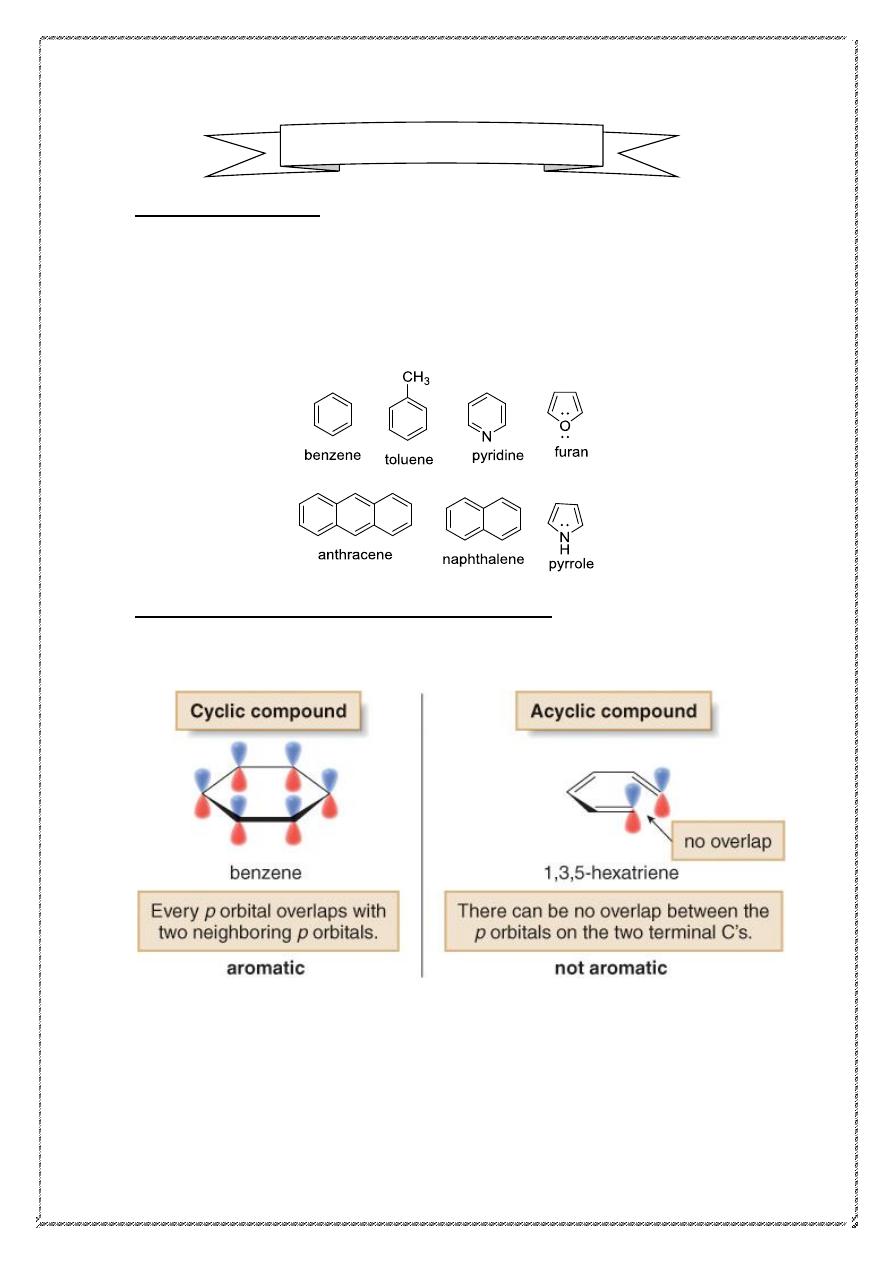
1
Aromatic compounds
Aromatic
compounds,
also
known
as arenes or aromatics,
are chemical compounds
that
contain conjugated planar
ring
systems with delocalized π-electrons clouds instead of discrete
alternating single and double bonds. Typical aromatic compounds
are benzene and toluene. They should satisfy Hückel's rule.
The Criteria for Aromaticity — Hückel’s Rule
Four structural criteria must be satisfied for a compound to be aromatic.
1-Amolecule must be cyclic.
To be aromatic, each p orbital must overlap with p orbitals on adjacent
atoms.
2-Amolecule must be planar
(
all atoms in the molecule lie in the same
plane)
3- Amolecule must be completely conjugated .
4-
The molecule has 4n+2π electrons (
Hückel’s rule)
Aromatic compounds
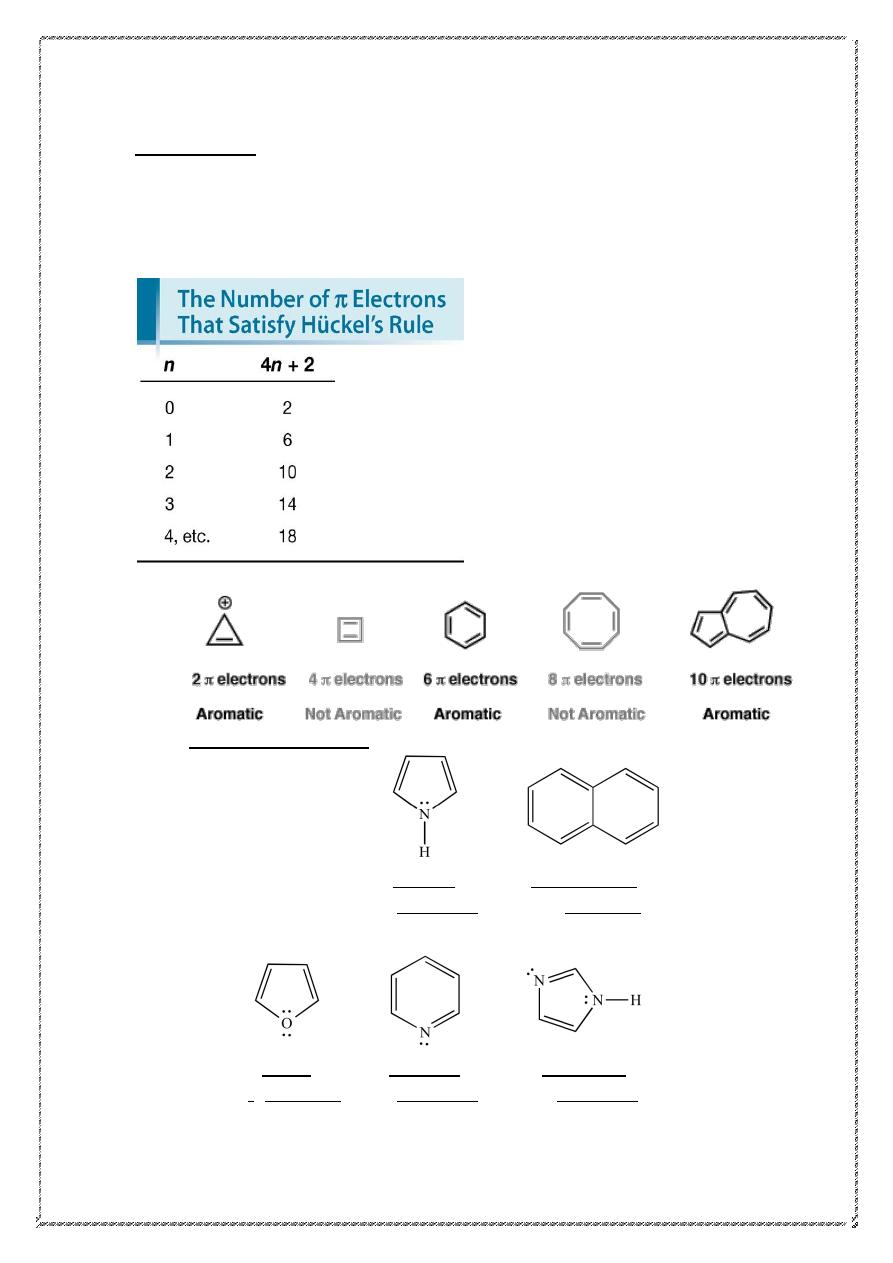
2
Hückel’s rule
A cyclic ring molecule follows Hückel's rule when the number of
its π-electrons equals 4n+2 where n is zero or any positive integer , The
most common case in six
electrons (n=1) which is found for example in
benzene ,pyrrole ,furan ,and pyridine .
6
n = 1
n = 2
electrons
n = 1
n = 1
n = 1
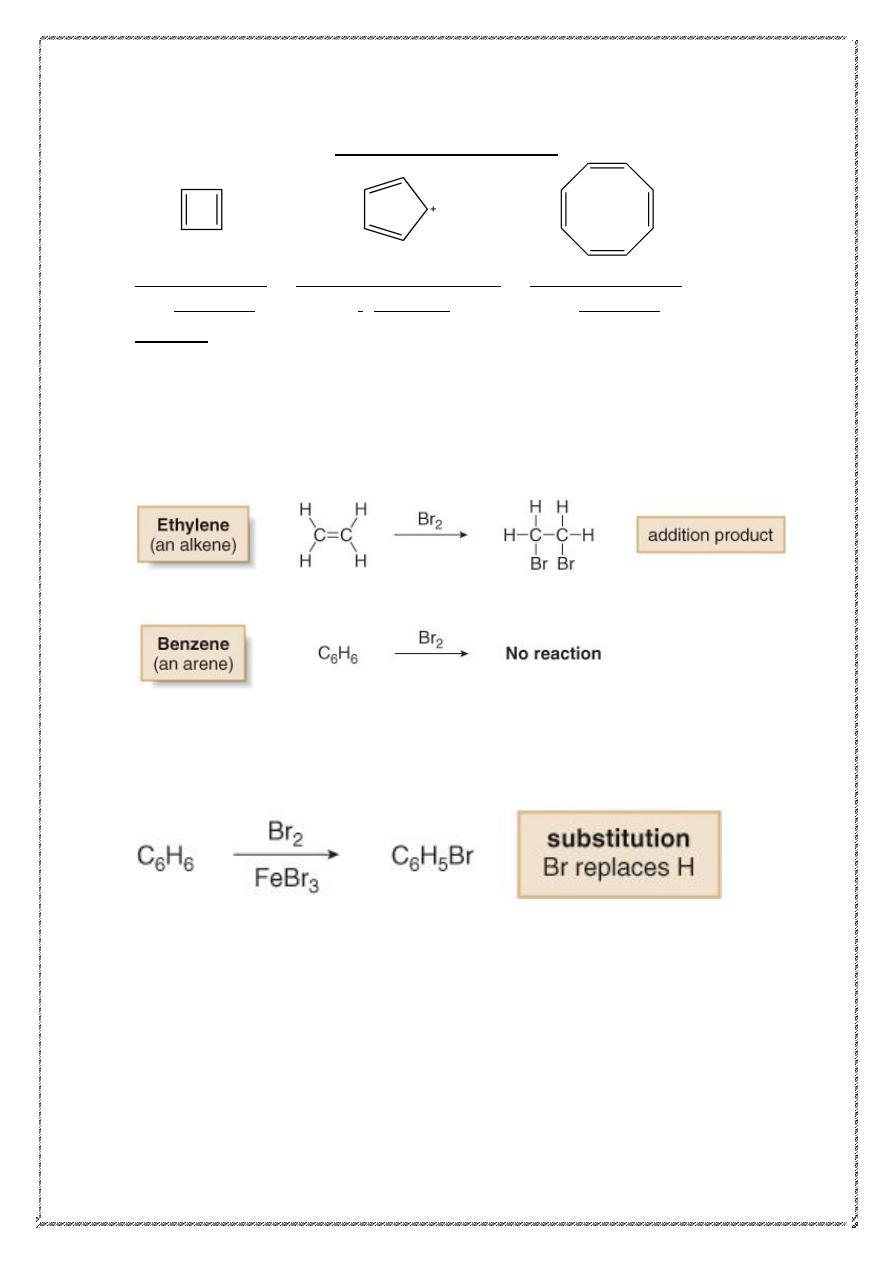
3
Benzene
• Benzene (C
6
H
6
) is the simplest aromatic hydrocarbon (or arene).
• Whereas unsaturated hydrocarbons such as alkenes, alkynes and
dienes readily undergo addition reactions, benzene does not.
• Benzene does react with bromine, but only in the presence of FeBr
3
(Lewis acid), and the reaction is a substitution, not an addition.
• Proposed structures of benzene must account for its high degree of
unsaturation and its lack of reactivity towards electrophilic
addition.
• August Kekulé proposed that benzene was a rapidly equilibrating
mixture of two compounds, each containing a six-membered ring
with three alternating
bonds.
• In the Kekulé description, the bond between any two carbon atoms
is sometimes a single bond and sometimes a double bond.
• These structures are known as Kekulé structures.
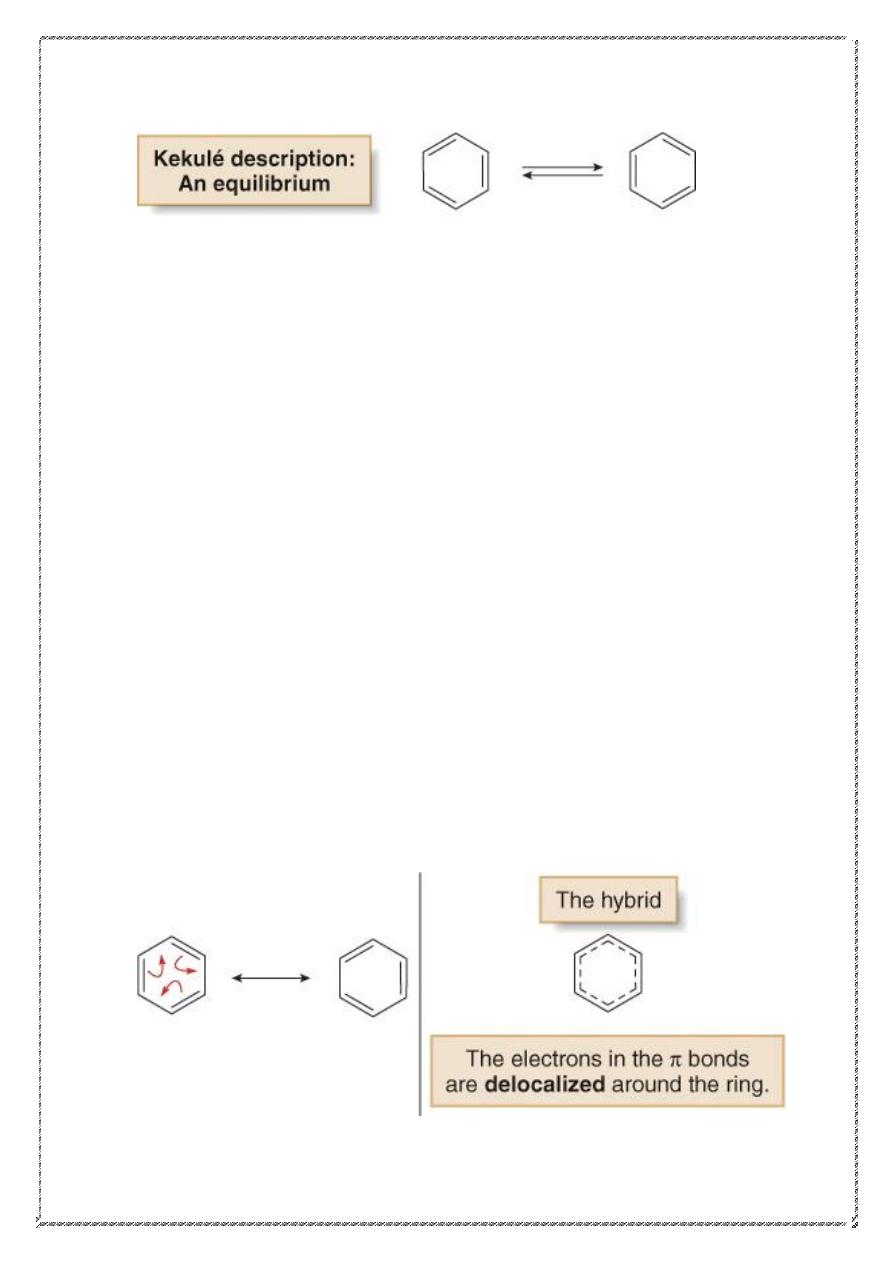
4
• Although benzene is still drawn as a six-membered ring with
alternating
bonds, in reality there is no equilibrium between the
two different kinds of benzene molecules.
• Current descriptions of benzene are based on resonance and
electron delocalization due to orbital overlap.
• In the nineteenth century, many other compounds having properties
similar to those of benzene were isolated from natural sources.
Since these compounds possessed strong and characteristic odors,
they were called aromatic compounds. It should be noted, however,
that it is their chemical properties, and not their odor, that make
them special.
Any structure for benzene must account for the following facts:
1. It contains a six-membered ring and three additional degrees of
unsaturation.
2. It is planar.
3. All C—C bond lengths are equal.
• The resonance description of benzene consists of two equivalent
Lewis structures, each with three double bonds that alternate with
three single bonds.
• The true structure of benzene is a resonance hybrid of the two
Lewis structures, with the dashed lines of the hybrid indicating the
position of the
bonds.
• We will use one of the two Lewis structures and not the hybrid in
drawing benzene. This will make it easier to keep track of the
electron pairs in the
bonds (the
electrons).
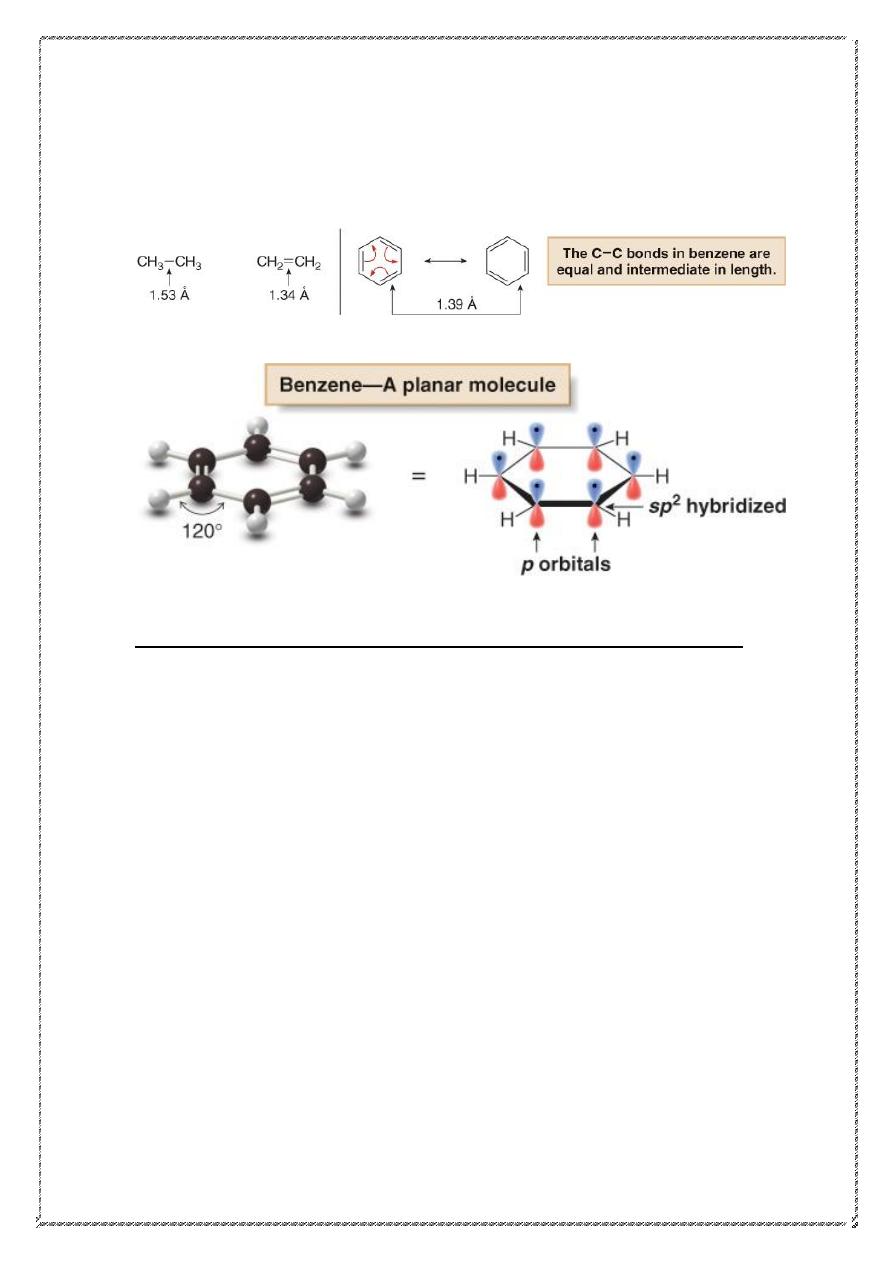
5
• In benzene, the actual bond length (1.39 Å) is intermediate
between the carbon—carbon single bond (1.53 Å) and the
carbon—carbon double bond (1.34 Å).
Stability of the benzene ring. Heats of hydrogenation and combustion:
Heats of hydrogenation and combustion of benzene are lower than
expected,
Heat of hydrogenation is the quantity of heat evolved when one mole
of an unsaturated compound is hydrogenated. In most cases the value is
about 28-30 kcal for each double bond the compound contains. It is not
surprising, then, that cyclohexene has a heat of hydrogenation of 28.6
kcal and cyclohexadiene has one about twice that (55.4 kcal.)We might
reasonably expect cyclohexatriene to have a heat of hydrogenation about
three times as large as cyclohexene, that is, about 85.8 kcal. Actually, the
value for benzene (49.8 kcal) is 36 kcal less than this expected amount.
This can be more easily visualized, perhaps, by means of an energy
diagram, in which the height of a horizontal line represents the potential
energy content of a molecule. The broken lines represent the expected
values, based upon three equal steps of 28.6 kcal. The final product,
cyclohexane, is the same in all three cases.
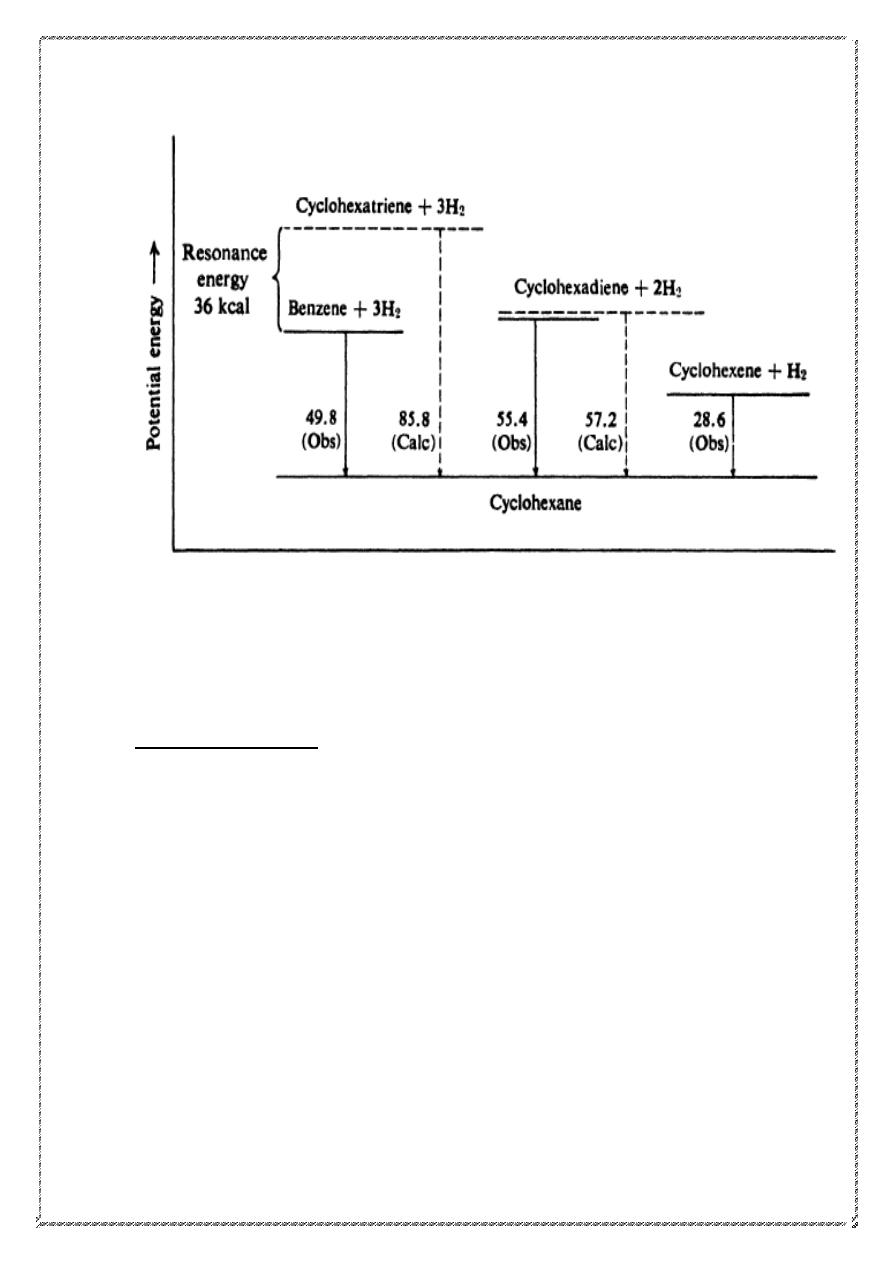
6
The fact that benzene evolves 36 kcal less energy than predicted can only
mean that benzene contains 36 kcal less energy than predicted; in other
words, benzene is more stable by 36 kcal than we would have expected
cyclohexatriene to be. The heat of combustion of benzene is also lower
than that expected, and by about the same amount.
Reactions of benzene
Benzene undergoes substitution rather than addition. Kekule's structure of
benzene is one that we would call "cyclohexatriene." We would expect
this cyclohexatriene, like the very similar compounds, cyclohexadiene
and cyclohexene, to undergo readily the addition reactions characteristic
of the alkene structure. As the examples in the following table show, this
is not the case; under conditions that cause an alkene to undergo rapid
addition, benzene reacts either not at all or very slowly.
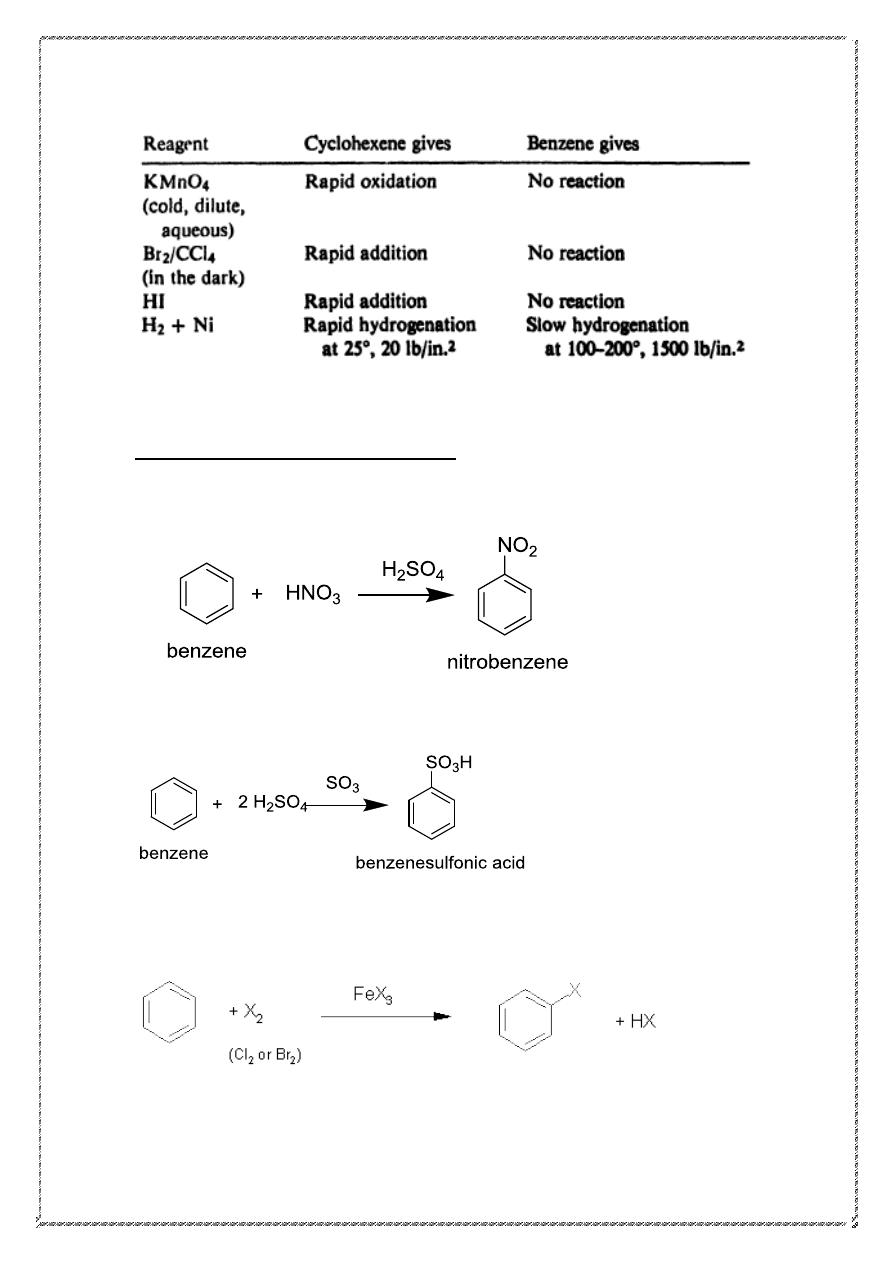
7
In place of addition reactions, benzene readily undergoes a new set of
reactions, all involving substitution. The most important are shown below
Electrophilic Aromatic Substitution
1. Nitration:
2. Sulfonation:
3. Halogenation:
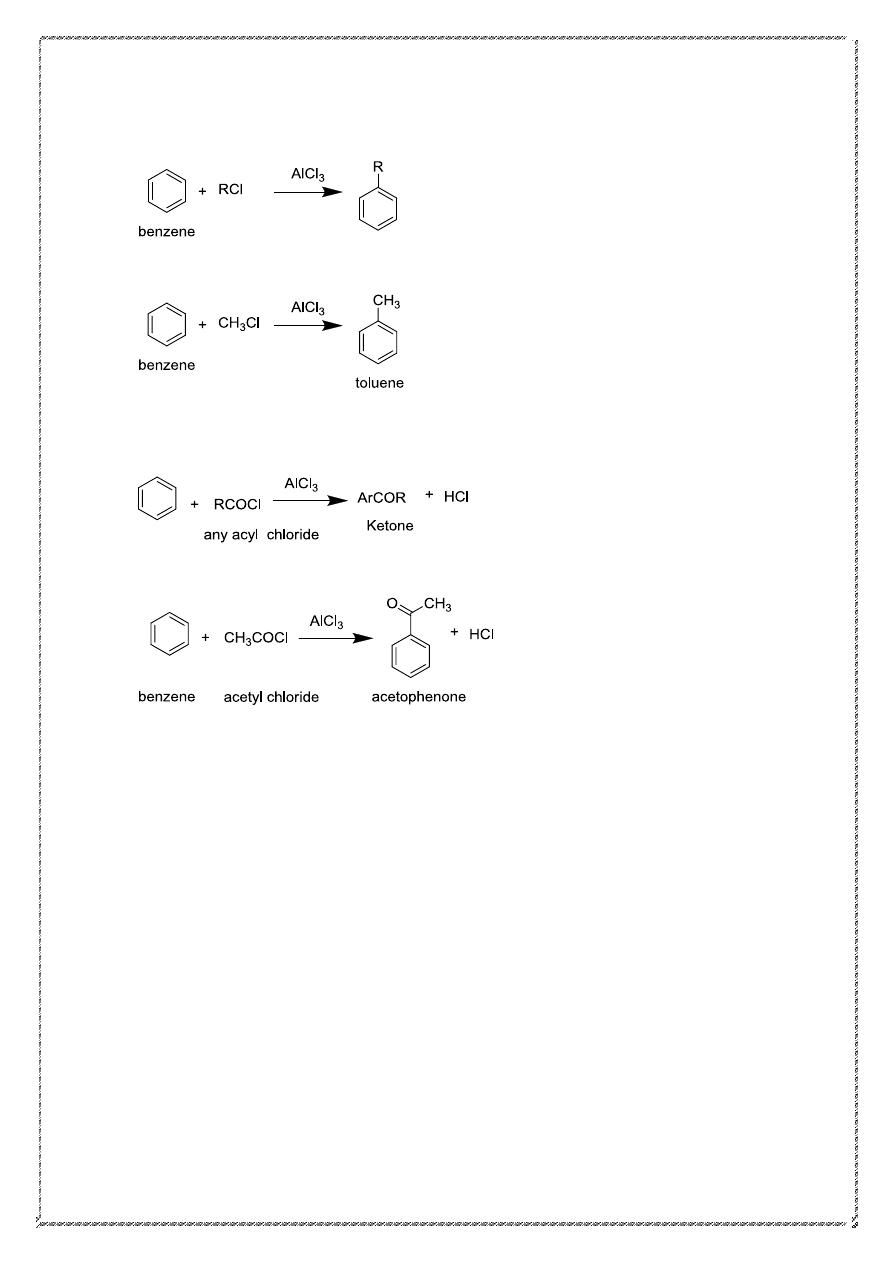
8
4. Friedel-Crafts alkylation:
5. Friedel-Crafts acylation:
In each of these reactions an atom or group has been substituted for one
of the hydrogen atoms of benzene. The product can itself undergo further
substitution of the same kind; the fact that it has retained the
characteristic properties of benzene indicates that it has retained the
characteristic structure of benzene. It would appear that benzene resists
addition, in which the benzene ring system would be destroyed, whereas
it readily undergoes substitution, in which the ring system is preserved.
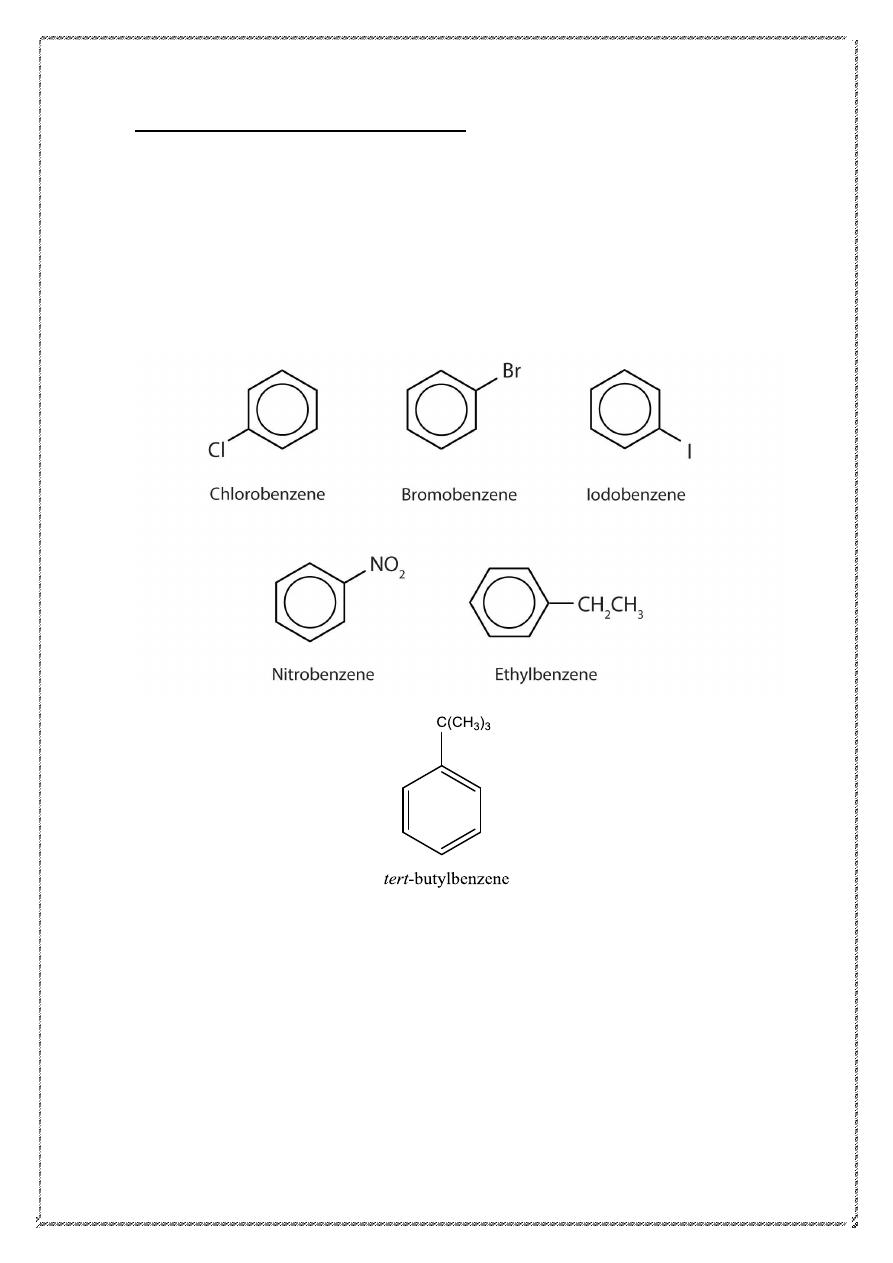
9
Nomenclature of benzene derivatives
All compounds that contain a benzene ring are aromatic, and substituted
derivatives of benzene make up the largest class of aromatic compounds.
Many such compounds are named by attaching the name of the
substituent as a prefix to benzene.
Many simple monosubstituted derivatives of benzene have common
names of long standing that have been retained in the IUPAC system.
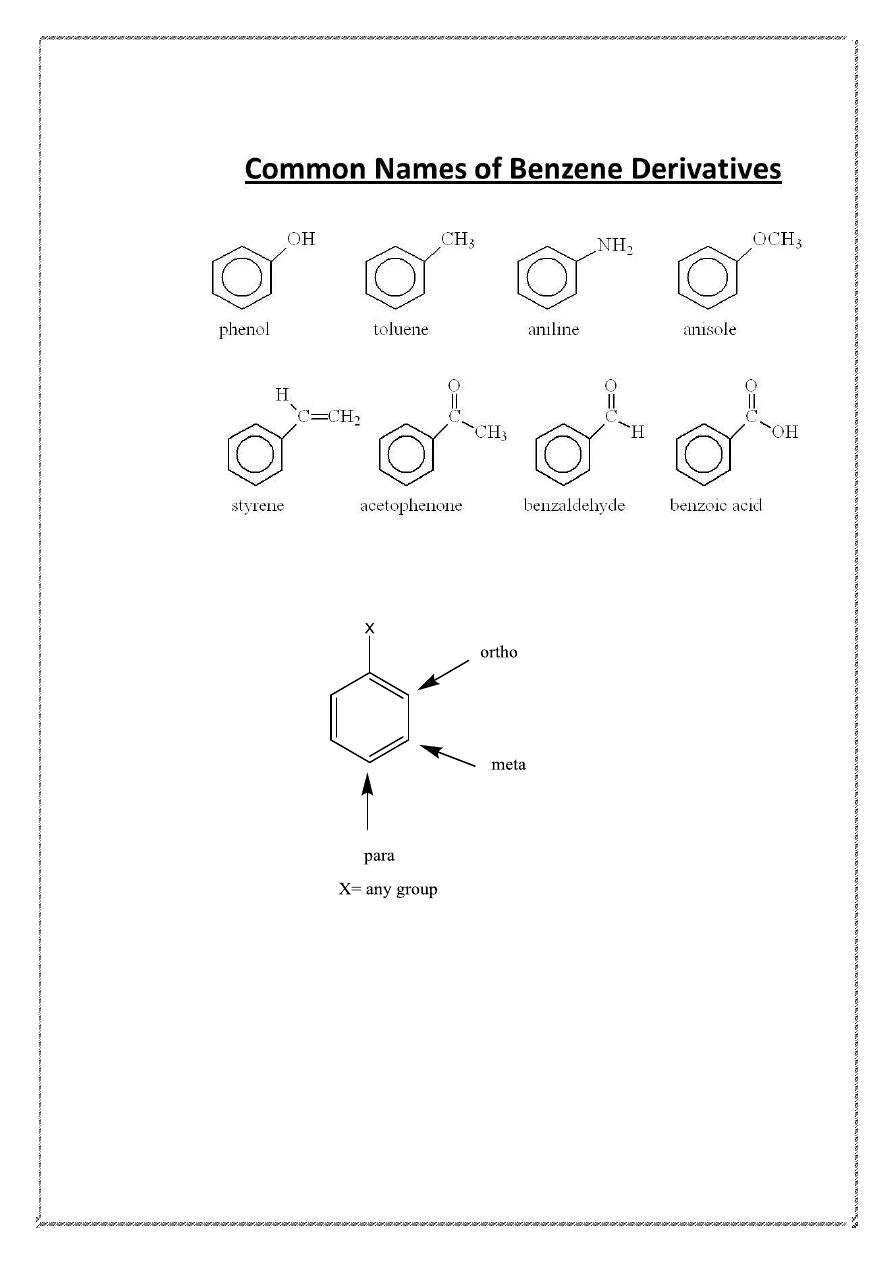
10
The prefix ortho signifies a 1,2-disubstituted benzene ring, meta signifies
1,3-disubstitution, and para signifies 1,4-disubstitution.
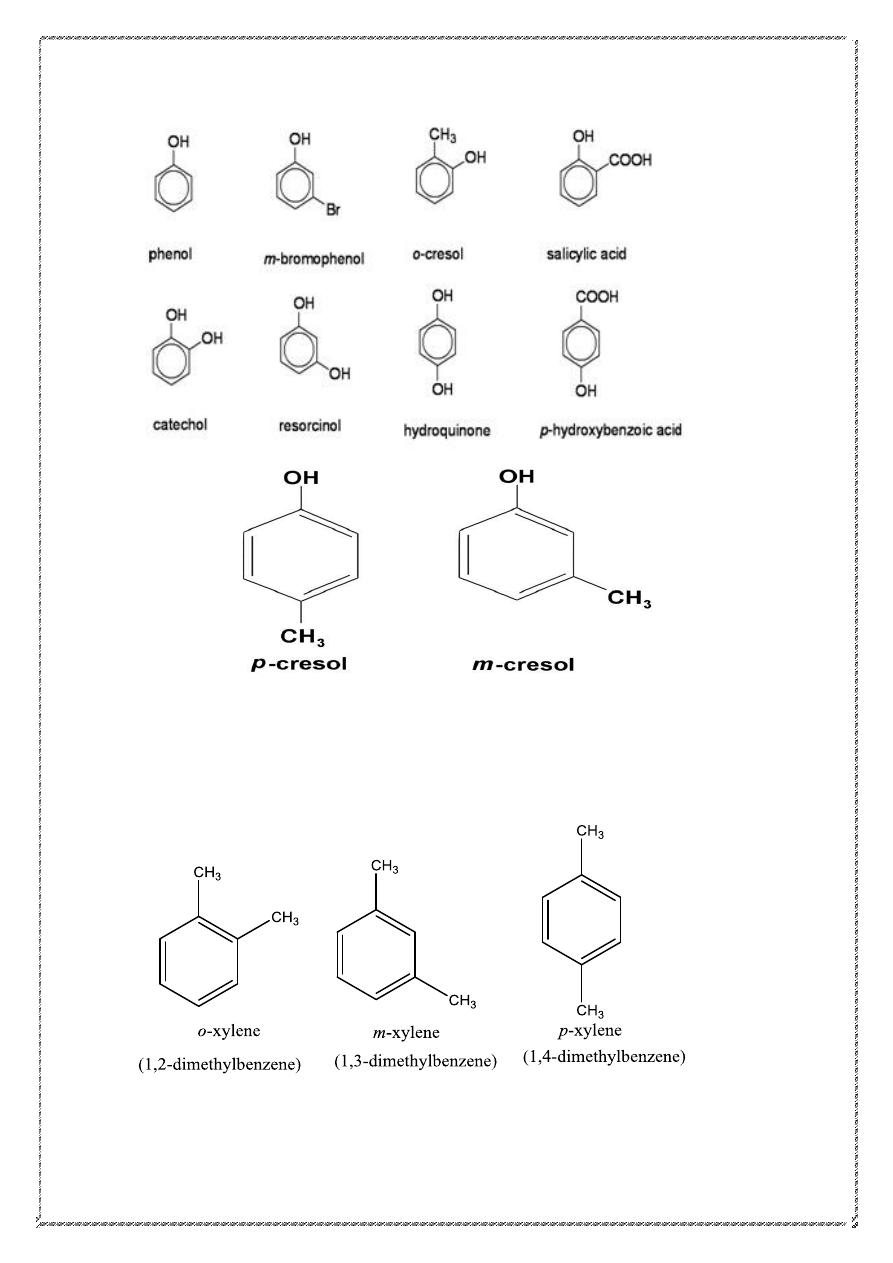
11
Dimethyl derivatives of benzene are called xylenes. There are three
xylene isomers, the ortho (o)-, Meta (m)-, and para (p) - substituted
derivatives.
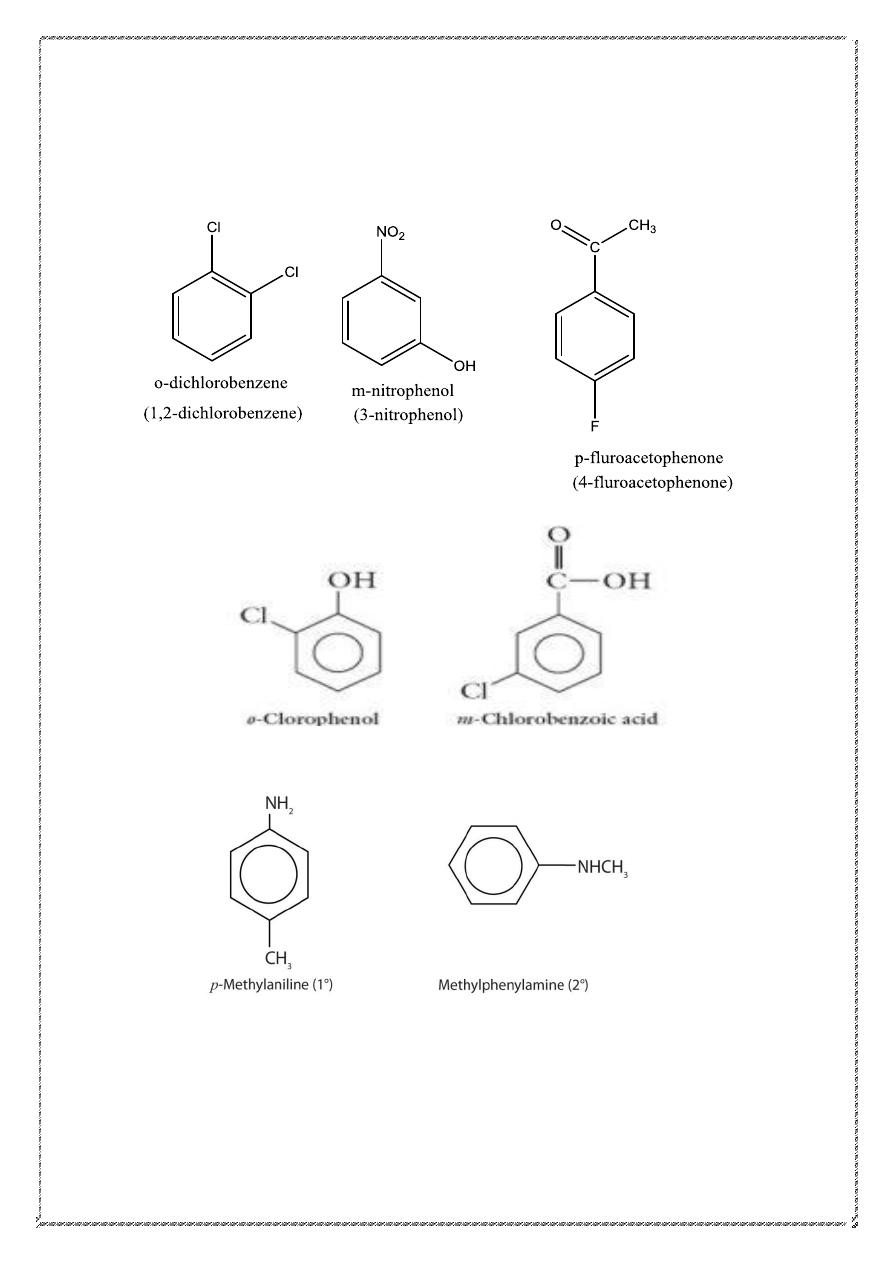
12
The prefixes o , m, and p can be used when a substance is named as a
benzene derivative or when a specific base name such as
The o, m, and p prefixes are not used when three or more substituents are
present on benzene; numerical locants must be used instead.
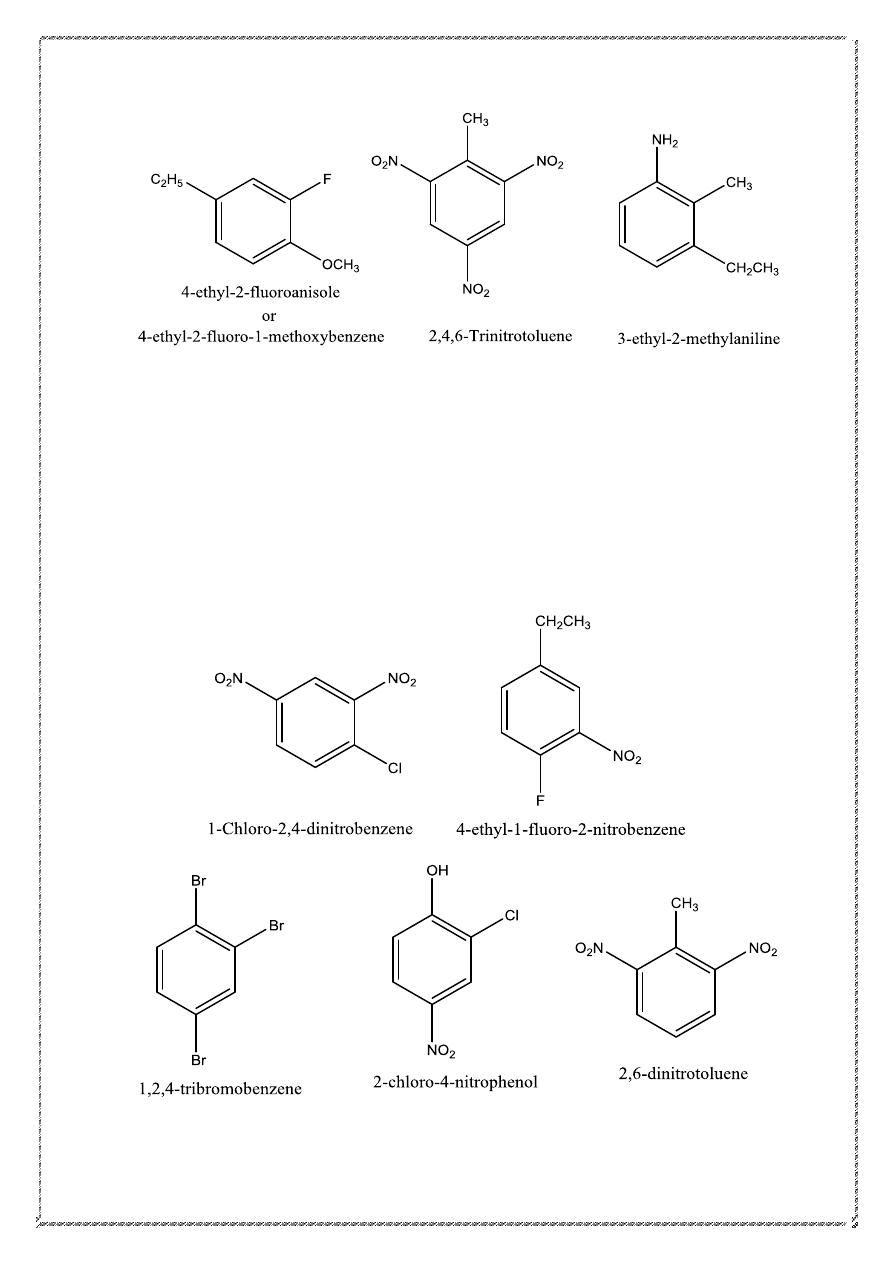
13
In these examples the base name of the benzene derivative determines the
carbon at which numbering begins: anisole has its methoxy group at C-l,
toluene its methyl group at C-l, and aniline its amino group at C-1. The
direction of numbering is chosen to give the next substituted position the
lowest number irrespective of what substituent it bears. The order of
appearance of substituents in the name is alphabetical. When no
simple base name other than benzene is appropriate, positions are
numbered so as to give the lowest locant at the first point of difference.
Thus, each of the following examples is named as a 1, 2, 4-trisubstituted
derivative of benzene rather than as a 1, 3, 4-derivative:
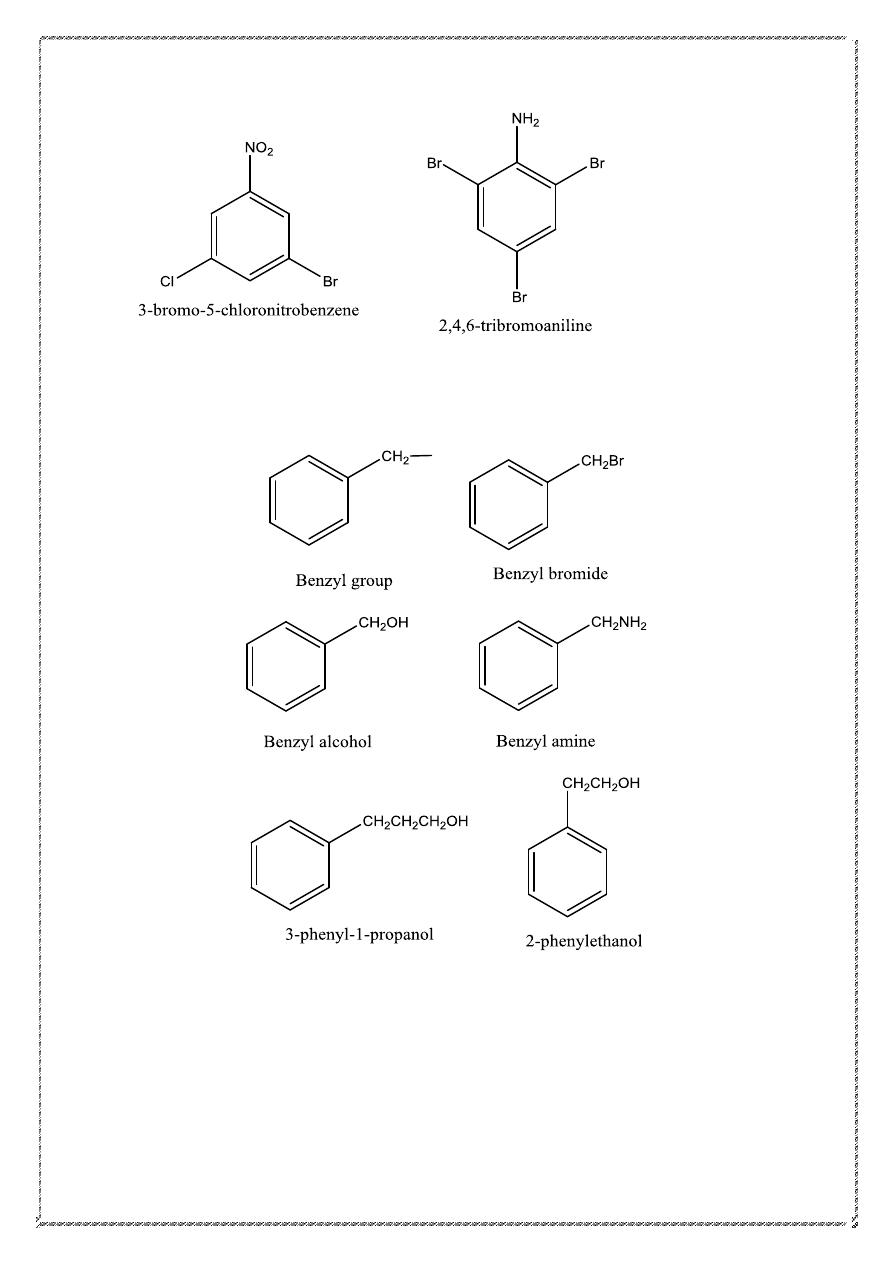
14
When the benzene ring is named as a substituent, the word phenyl stands
for C
6
H
5
–. Similarly, an arene named as a substituent is called an aryl
group. A benzyl group is C
6
H
5
CH
2
- .
Biphenyl is the accepted IUPAC name for the compound in which two
benzene rings are connected by a single bond.
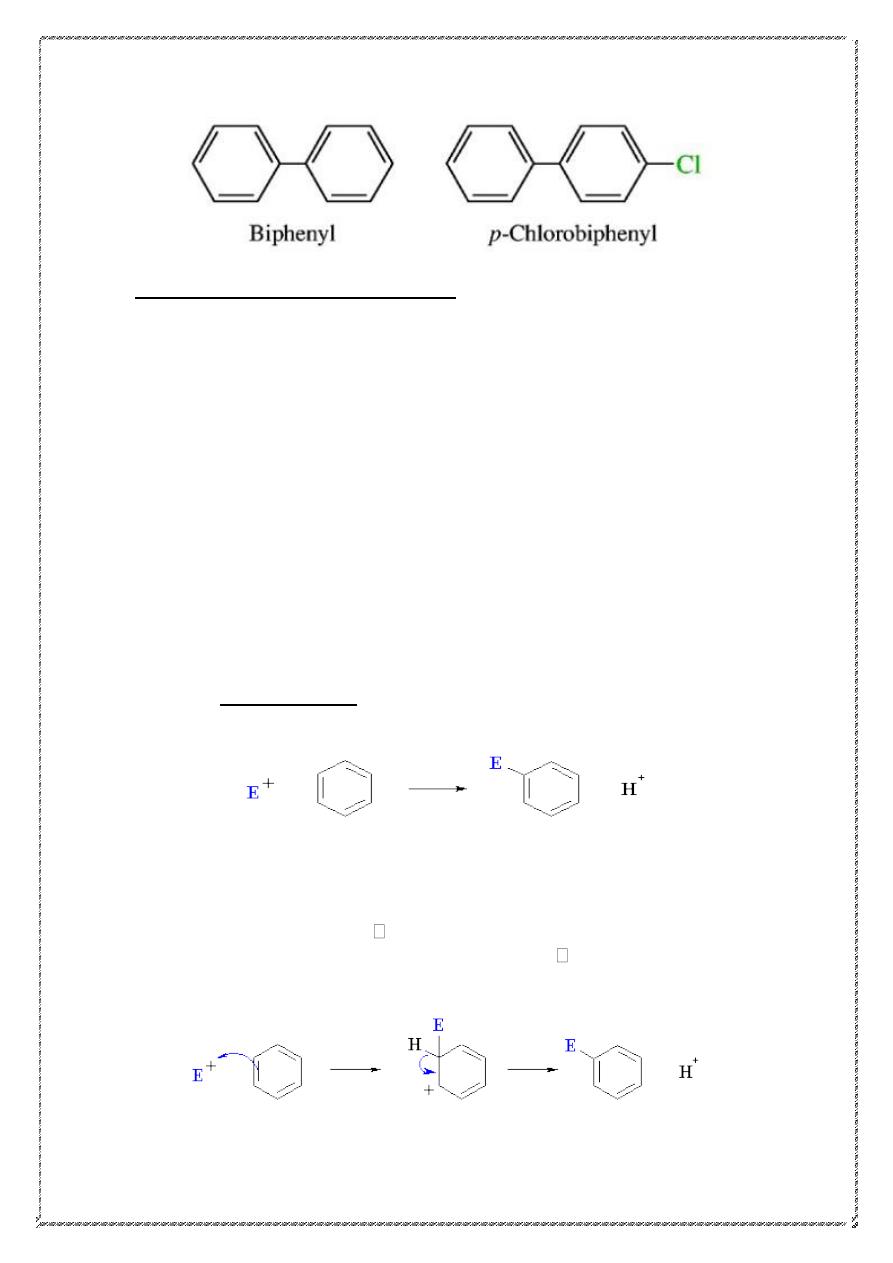
15
Electrophilic Aromatic Substitution
It is not surprising that in its typical reactions the benzene ring serves as a
source of electrons, that is, as a base. The compounds with which it reacts
are deficient in electrons, that is, are electrophilic reagents or acids. Just
as the typical reactions of the alkenes are electrophilic addition reactions,
so the typical reactions of the benzene ring are electrophilic substitution
reactions. Electrophilic aromatic substitution includes a wide variety of
reactions: nitration, halogenation, sulfonation, and Friedel-Crafts
reactions, undergone by nearly all aromatic rings; reactions like
nitrosation and diazo coupling, undergone only by rings of high
reactivity.
Electrophilic Aromatic Substitution (EAS) is a substitution reaction
usually involving the benzene ring; more specifically it is “a reaction in
which the hydrogen atom of an aromatic ring is replaced as a
consequence of electrophilic attack on the aromatic ring.”
An electrophile, E
+
, is an electron poor species that will react with
an electron rich species.
There are three fundamental components to an electrophilic substitution
reaction:
1. formation of the new bond from a C=C in the arene nucleophile
2. removal of the proton by breaking the C-H bond
3. reform the C=C and restore aromaticity
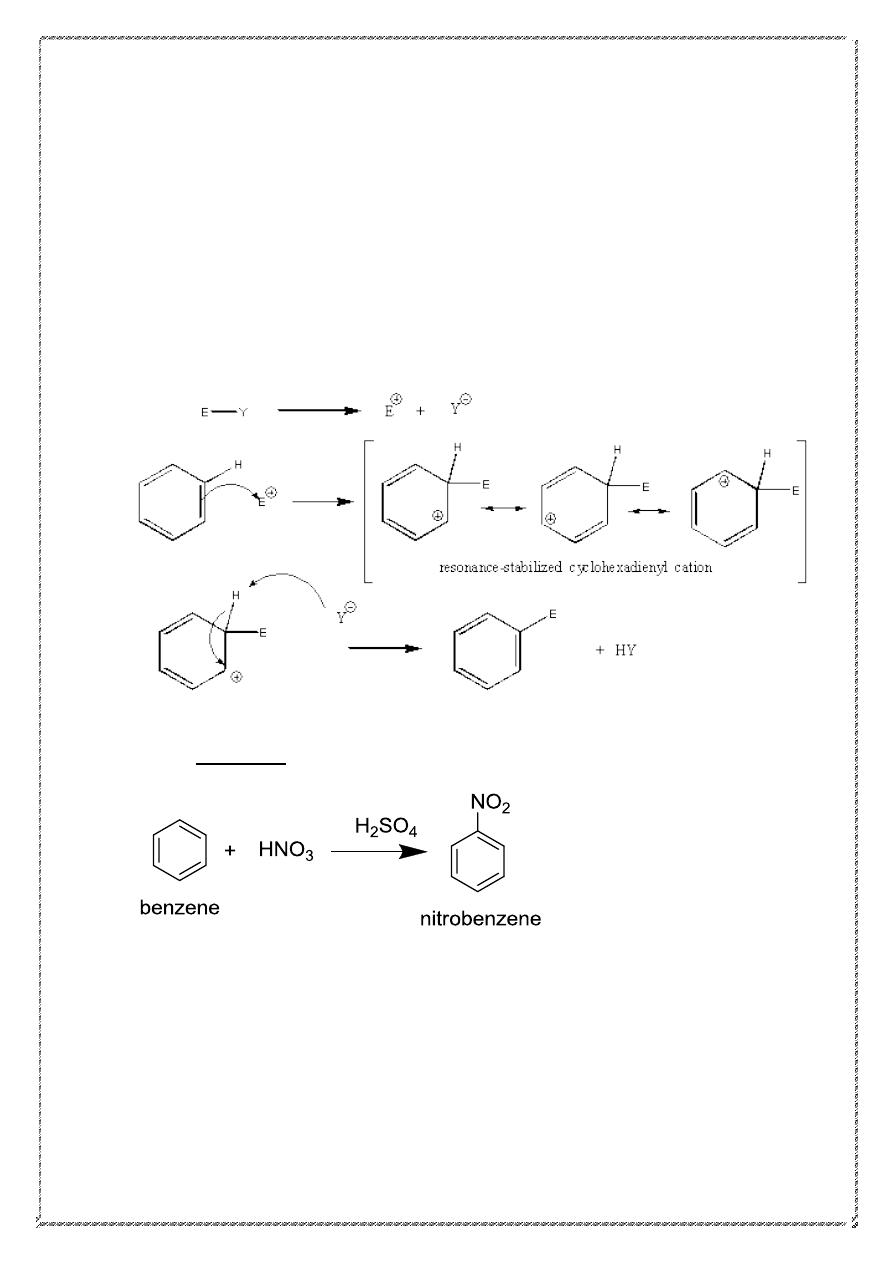
16
The mechanism is represented by the following series of events:
Formation of the reactive electrophile, E
+
Slow reaction of the arene C=C with the E
+
to give a resonance
stabilized carbocation (see below)
Loss of H
+
from the carbocation to restore the C=C and the
aromatic system
The reaction of the electrophile E
+
with the arene is the slow step since it
results in the loss of aromaticity even though the resulting cation is still
resonance stabilized.
1. Nitration:
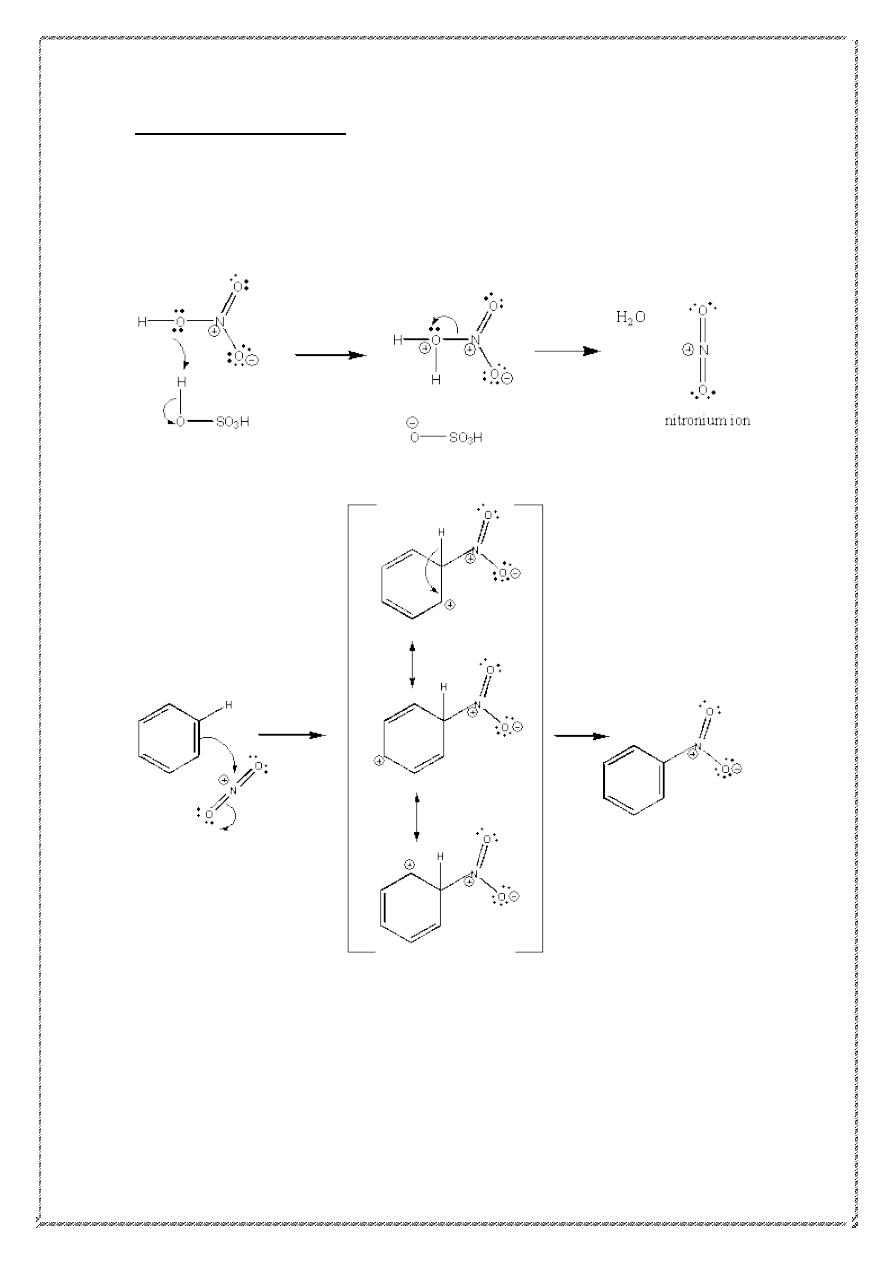
17
Mechanism of nitration
1) Nitration of an aromatic is accomplished by first obtaining a
reactive electrophile NO
2
+
and then combining it with an
aromatic :
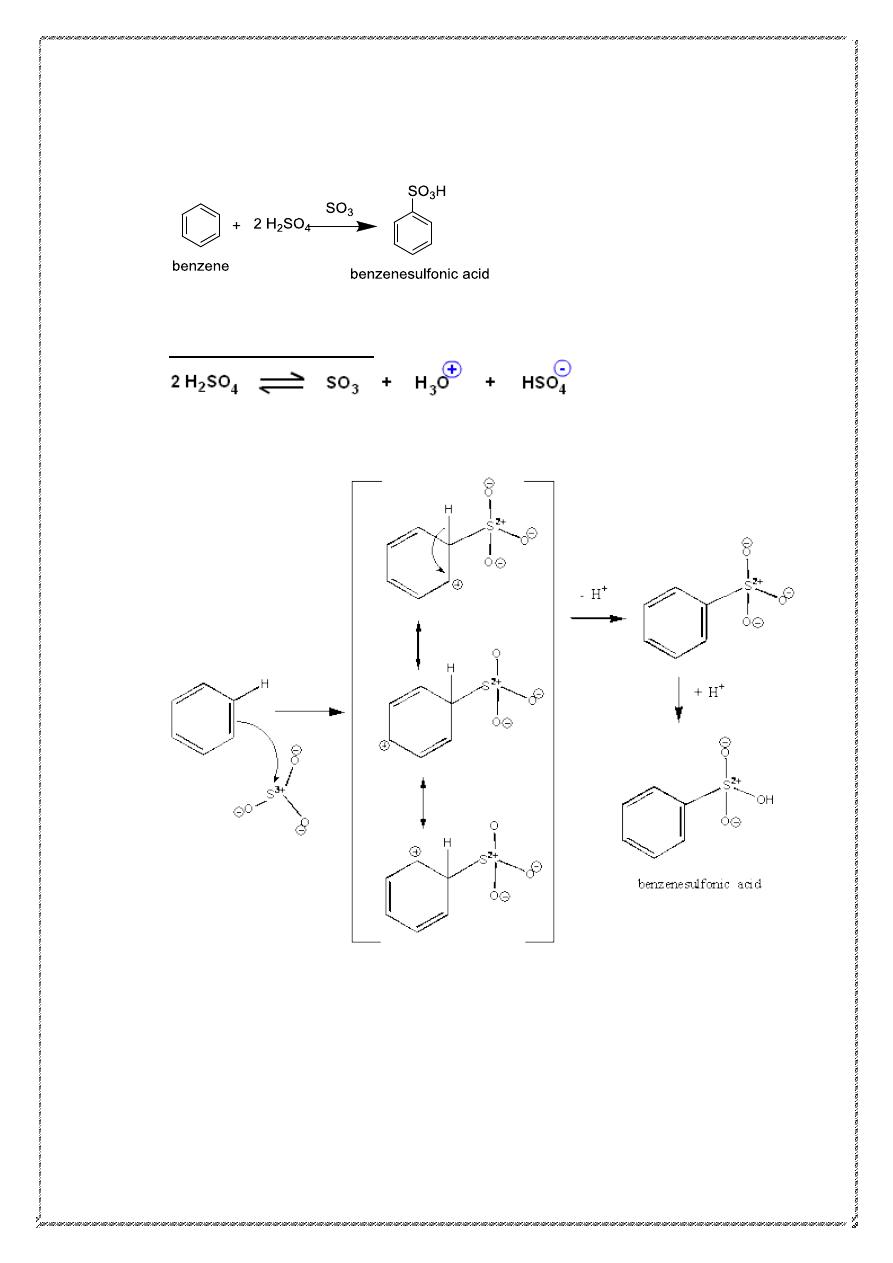
18
2 .Sulfonation:
Mechanism of reaction
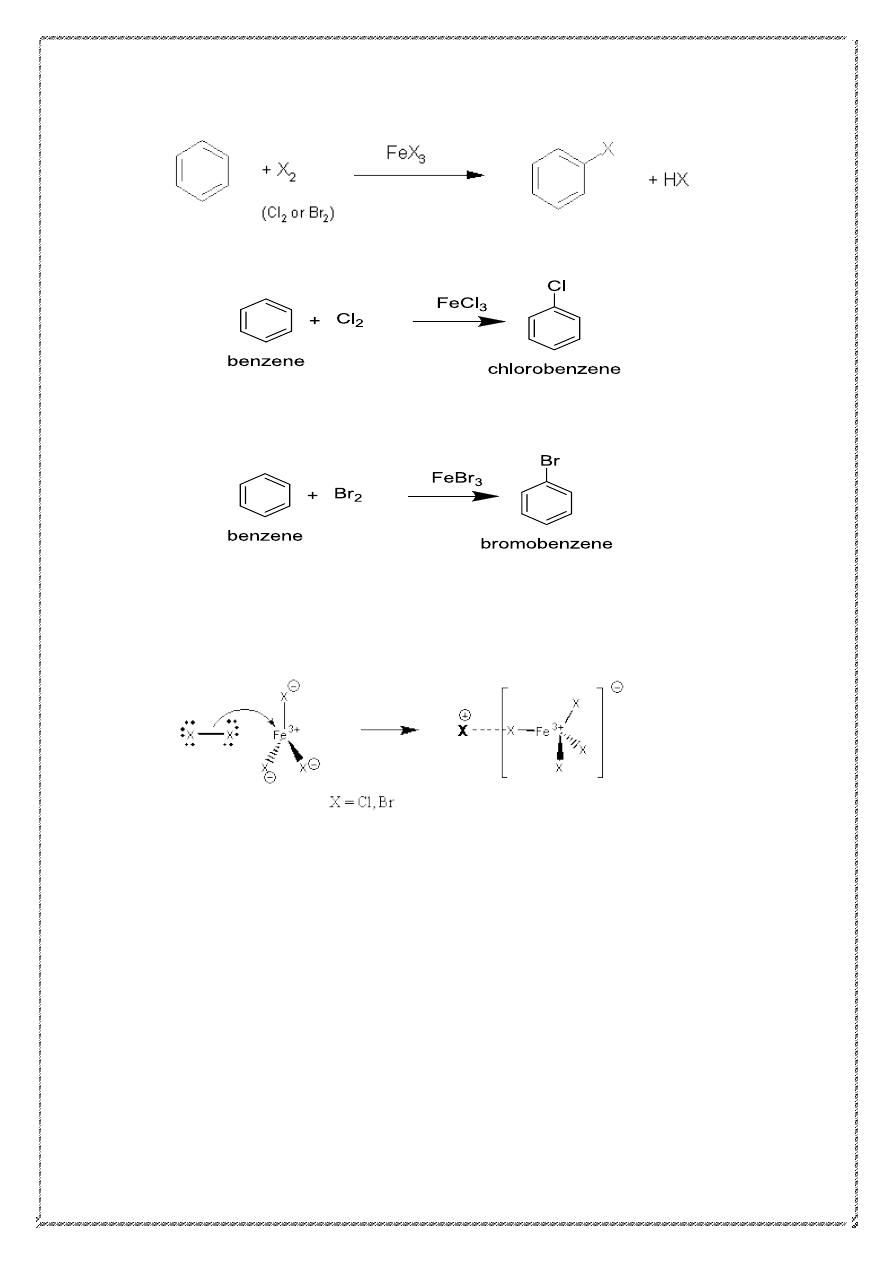
19
2) halogenation
Mechanism Of halogenation
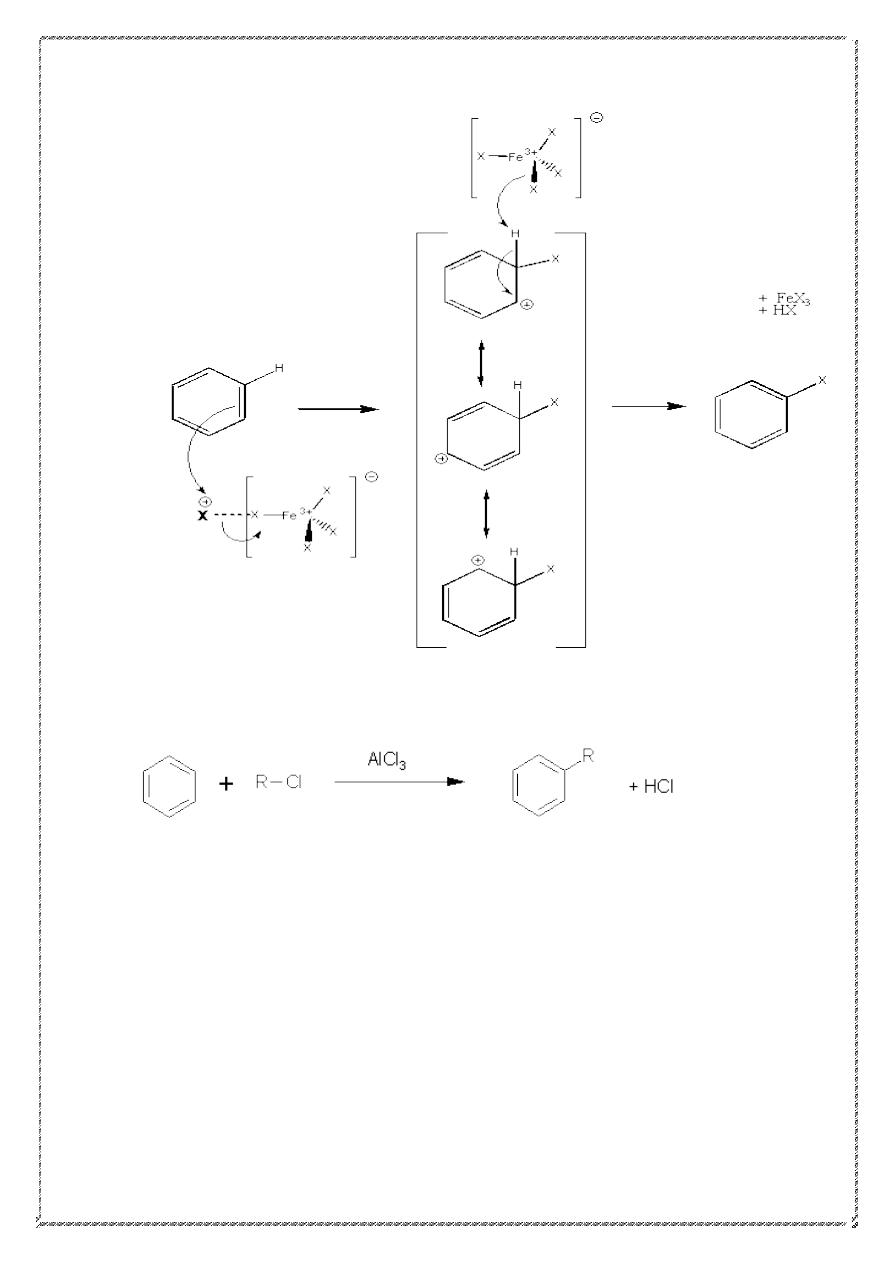
20
3) Friedel-Crafts Alkylation
R = 2
o
, 3
o
, -CH
3
, -CH
2
CH
3
Primary alkyl groups other than Me or Et rearrange.
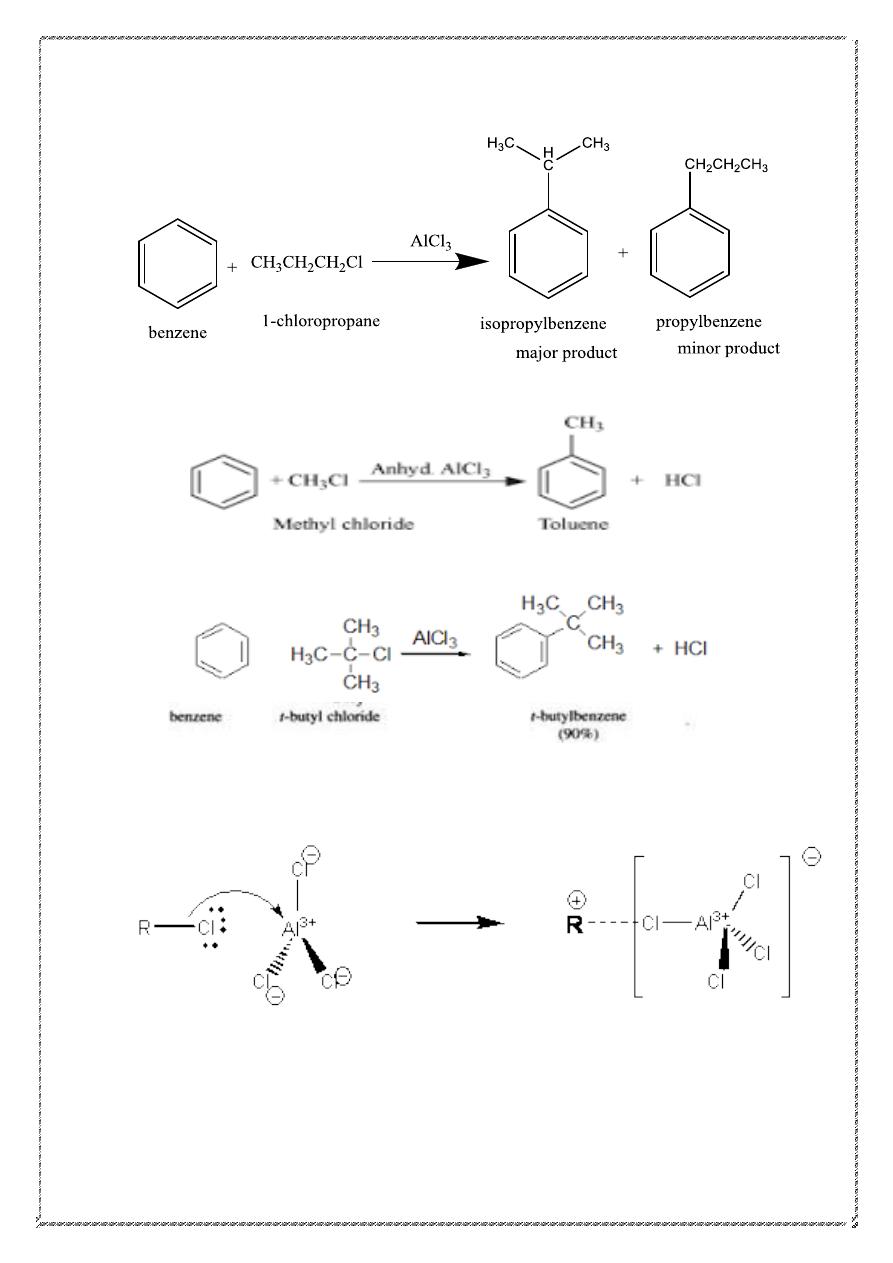
21
Mechanism of alkylation
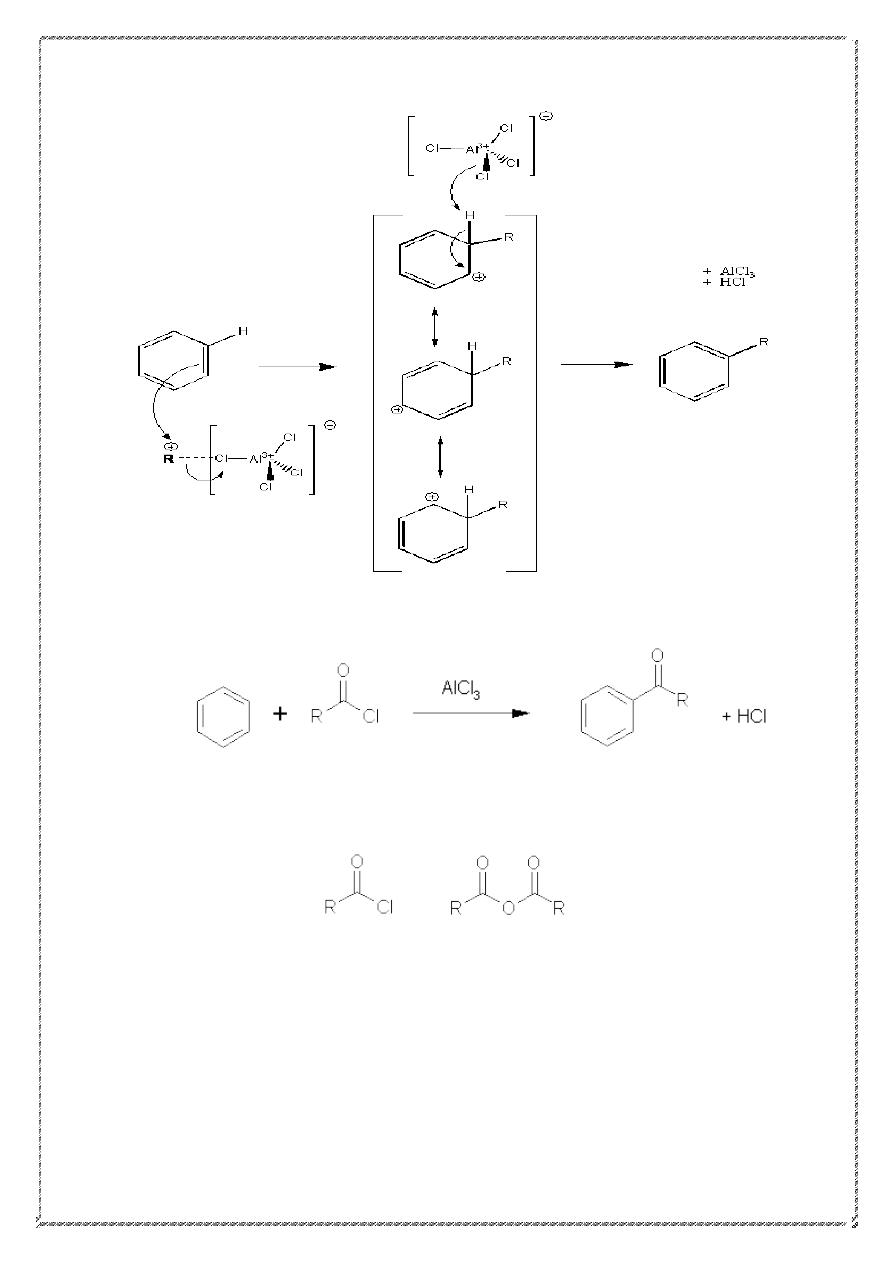
22
4) Friedel-Crafts Acylation
.
R = alkyl or aryl group.
This reaction works with acid chlorides (or acyl) or acid
anhydrides.
Acid chloride acid anhydride
An important feature of this reaction: no rearrangements occur. The
mechanism involves the formation of the acylium ion (acyl cation).the
stability of this ion (due to resonance stabilization) avoids rearrangement.
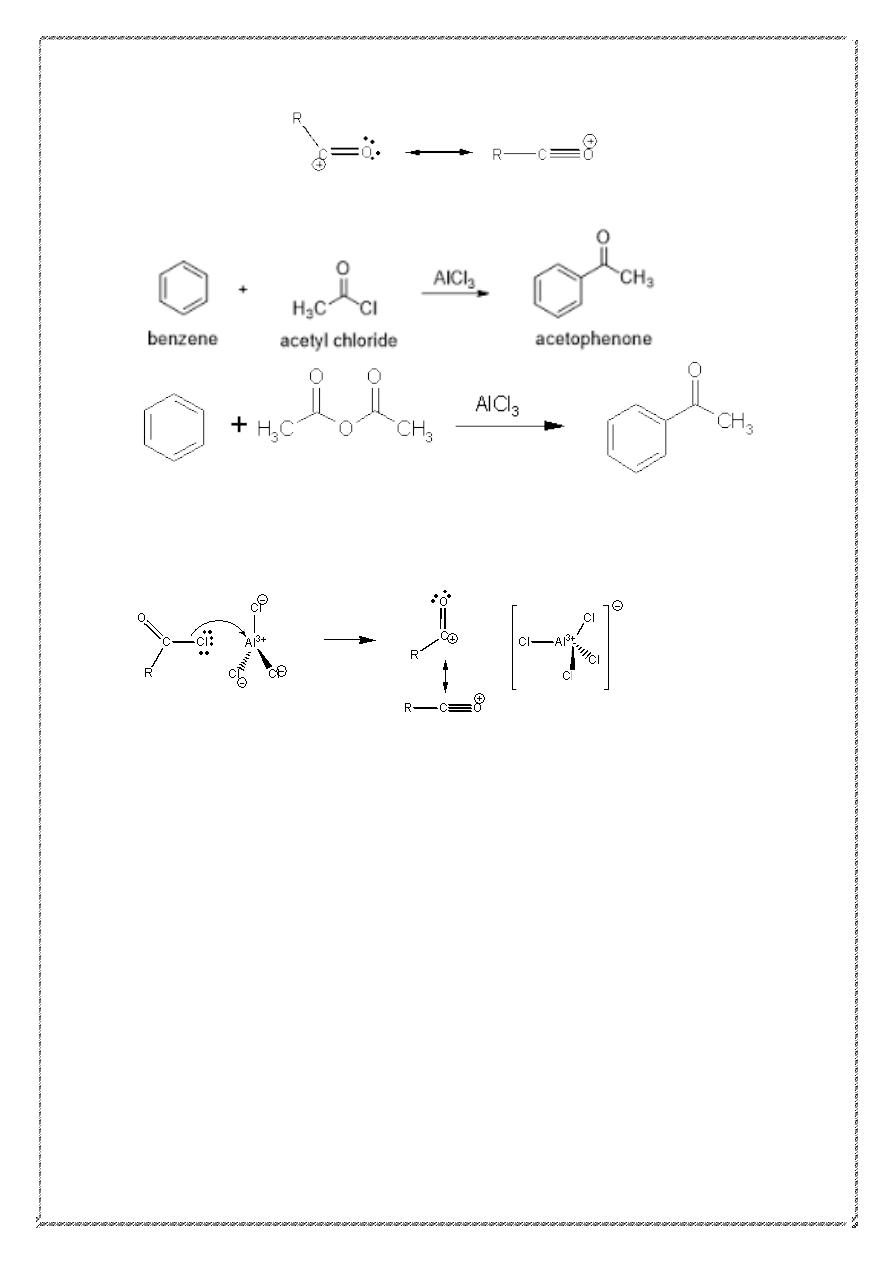
23
Acylium ion (acyl cation)
Mechanism of acylation:-
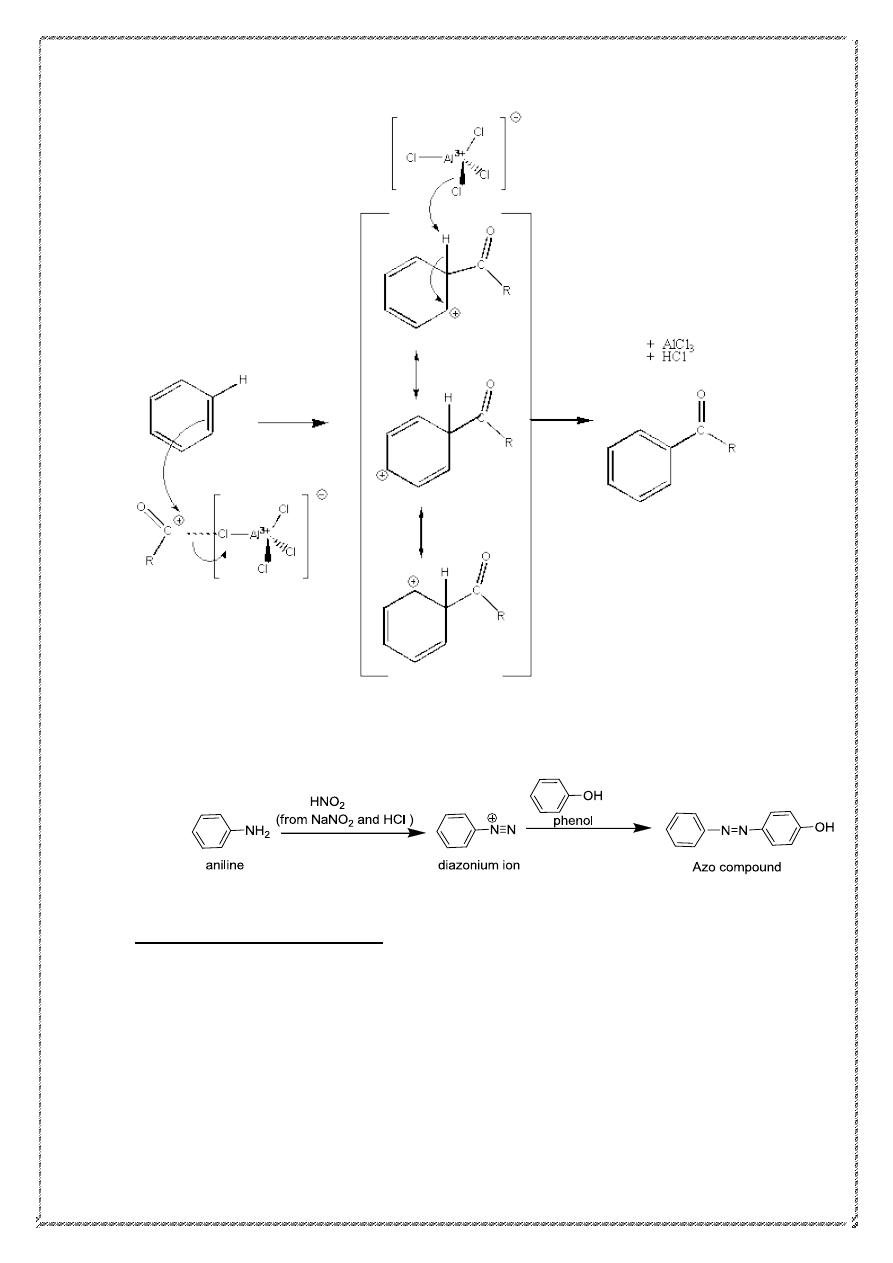
24
5). Diazo coupling:
Effect of substituent groups
Like benzene, toluene undergoes electrophilic aromatic substitution:
sulfonation, for example. Although there are three possible mono
sulfonation products, this reaction actually yields appreciable amounts of
only two of them: the o- and p- isomers.
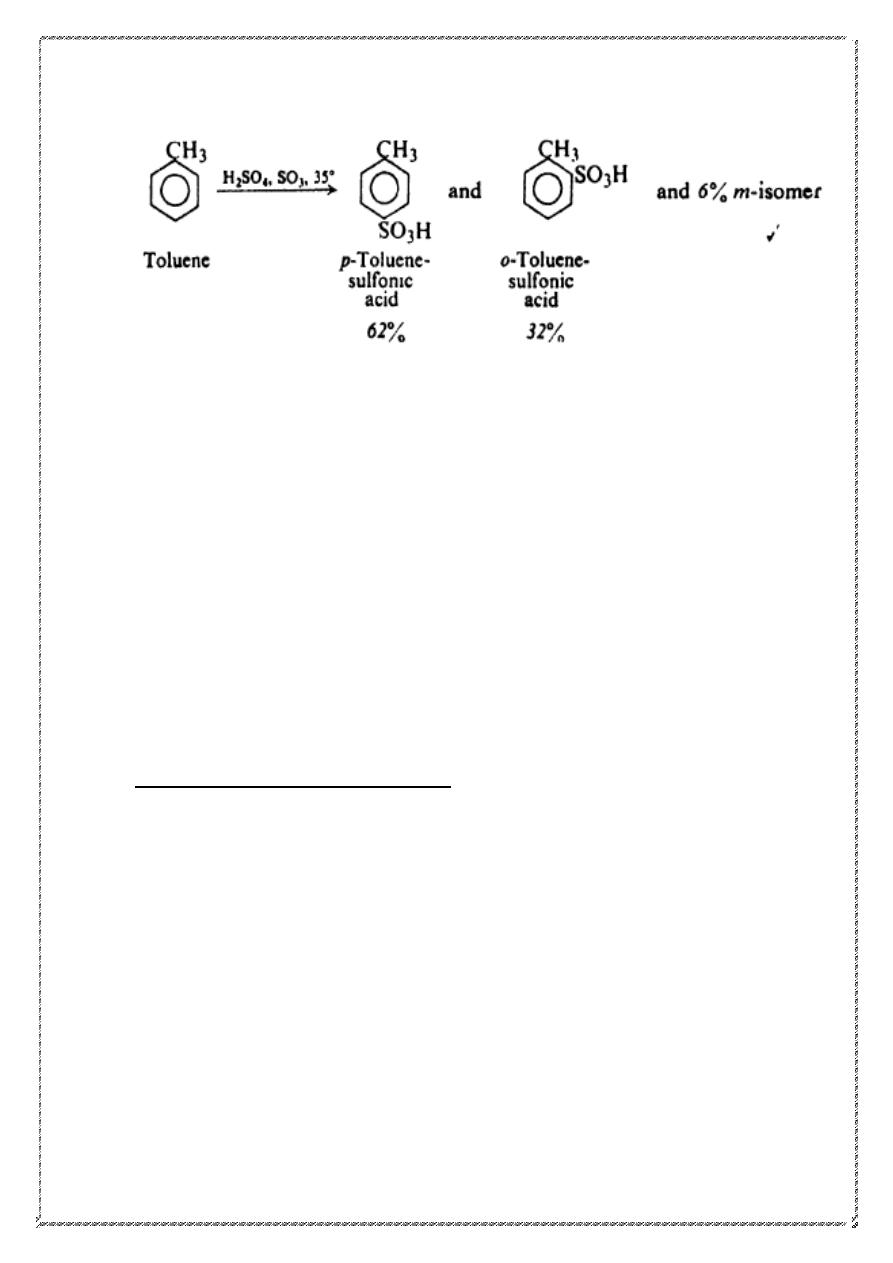
25
On the other hand, nitrobenzene, to take a different example, has been
found to undergo substitution more slowly than benzene, and to yield
chiefly the meta isomer. Like methyl or nitro, any group attached to a
benzene ring affects the reactivity of the ring and determines the
orientation of substitution. When an electrophilic reagent attacks an
aromatic ring, it is the group already attached to the ring that determines
how readily the attack occurs and where it occurs.
A group that makes the ring more reactive than benzene is called an
activating group.
A group that makes the ring less reactive than benzene is called a
deactivating group.
A group that causes attack to occur chiefly at positions ortho and para to
it is called an ortho , para director.
A group that causes attack to occur chiefly at positions Meta to it is called
A Meta director.
Classification of substituent groups
Nearly all groups fall into one of two glasses: activating and ortho, para-
directing, or deactivating and meta-directing. The halogens are in a class
by themselves, being deactivating but ortho-, para- directing.
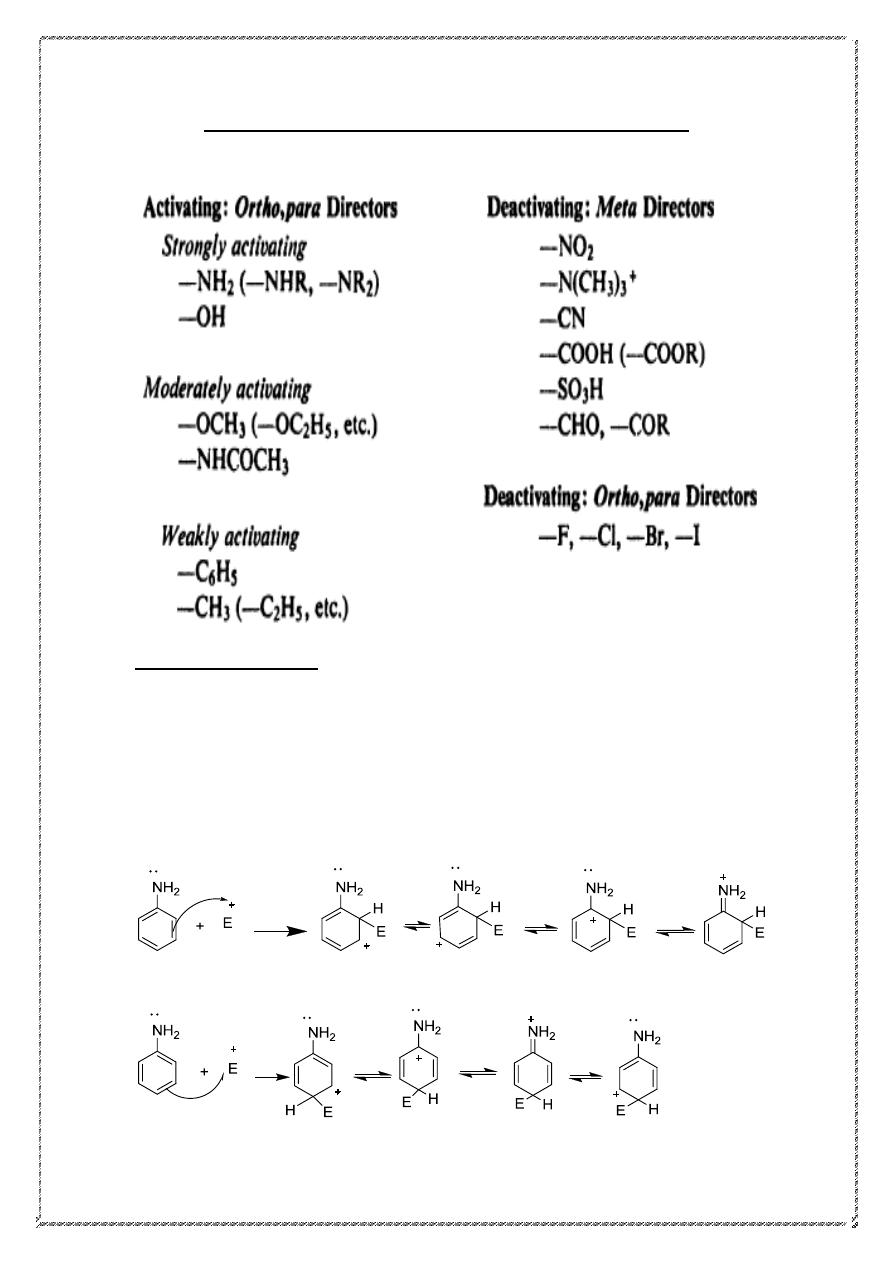
26
Effect of groups on electrophilic aromatic substitution
Ortho/para directors
Groups with unshared pairs of electrons, such as the amino group
of aniline,
are
strongly activating and ortho/para-directing.
Such activating groups donate those unshared electrons to the pi system.
When the electrophile attacks the ortho and para positions of aniline,
the nitrogen atom can donate electron density to the pi system (forming
an iminium ion), giving four resonance structures This substantially
enhances the stability of the cationic intermediate.
ortho
Para
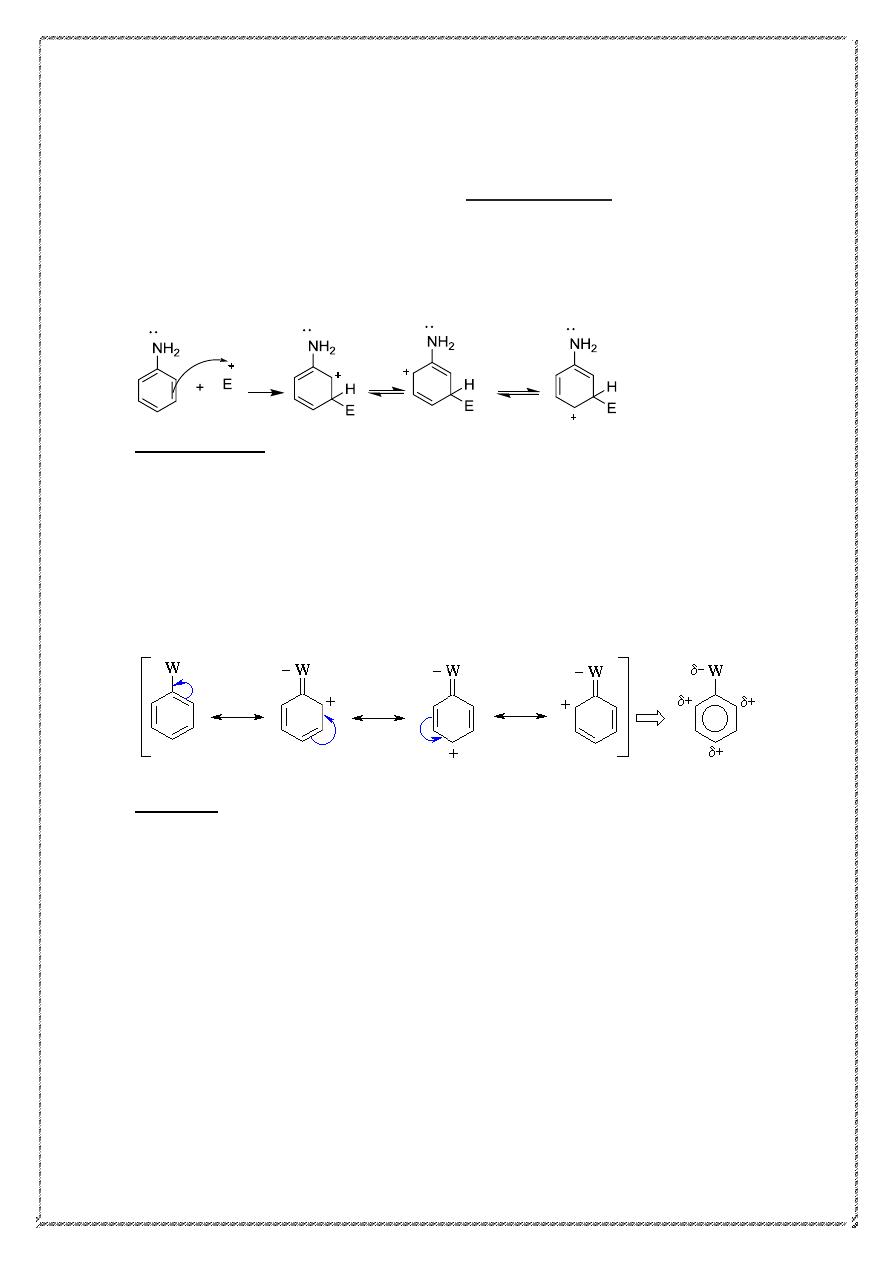
27
Compare this with the case when the electrophile attacks
the Meta position. In that case, the nitrogen atom cannot donate electron
density to the pi system, giving only three resonance contributors. For
this reason, the meta-substituted product is produced in much smaller
proportion to the ortho and para products.
Meta
Meta directors
Electron withdrawing groups (EWG) with pi bonds to electronegative
atoms (e.g. -C=O, -NO
2
) adjacent to the pi system deactivate the
aromatic ring by decreasing the electron density on the ring through
a resonance withdrawing effect. The resonance only decreases the
electron density at the ortho- and para- positions. Hence these sites
are less nucleophilic, and so the system tends to react with electrophiles
at the meta sites.
Halogens: - Are a special case since they are deactivating groups yet
are ortho /para directors.
1) They are deactivating due to their high electronegativities (inductive
effects).
2) They are ortho/para directors since they can, via their lone pairs,
donate electrons into the pi system of the ring when substitution is o/p
(resonance effect)
3) The high electronegativity of halogen atoms leads to overall ring
deactivating by their electron-withdrawing inductive effect .However
,their electro-donating resonance effect causes substitution to be more
favorable ortho and para positions than at meta positions
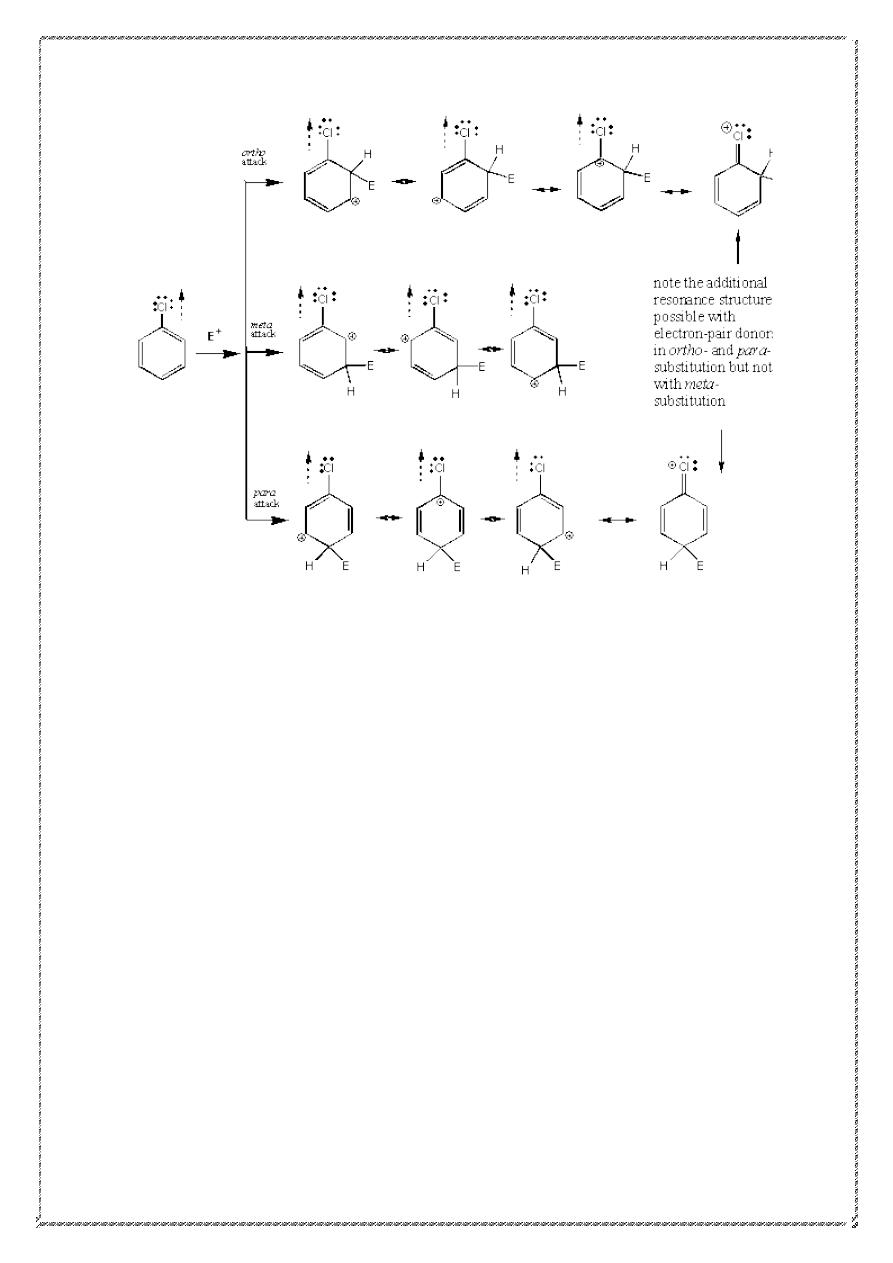
28